When Nicholas Bellono brought a live California two-spot octopus back to his lab for the first time, he was nervous. Octopuses are highly intelligent. They’re also known to escape through the tiniest openings. “I just watched it for a bit,” he remembers. “It’s crazy, all the stuff that they do with their arms.” The 35-year-old associate professor of biology wondered what those arms were doing. “I looked them over to see if I could find sensory cells. I thought, maybe they detect light to help the animals know how to change their skin patterns for camouflage, or maybe they sense chemicals.”
A concerted research effort led by Bellono yielded the discovery that the suckers on the long arms are loaded with receptors that let octopuses taste what they touch. This allows them to probe dark and narrow crevices on the seafloor to locate and identify prey. The scientists also found that the sucker receptors had evolved from an ancestral cell type—but differently in octopuses than in their cephalopod cousins, the squid. “[Squid] don’t do exploration with their arms,” Bellono explains. Rather, they hunt visually, ensnaring prey with two long, hooked tentacles, which they use to draw their food within range of their eight arms and beak. Then they use their arms to taste what they have caught, deciding whether to accept or reject it as food. Bellono found that these ambush predators have more receptors that can sense water-soluble chemicals from their prey, particularly bitter tastes, and far fewer of the hydrophobic sensory cells found in octopus suckers that require physical contact to sense any object’s chemical signature.
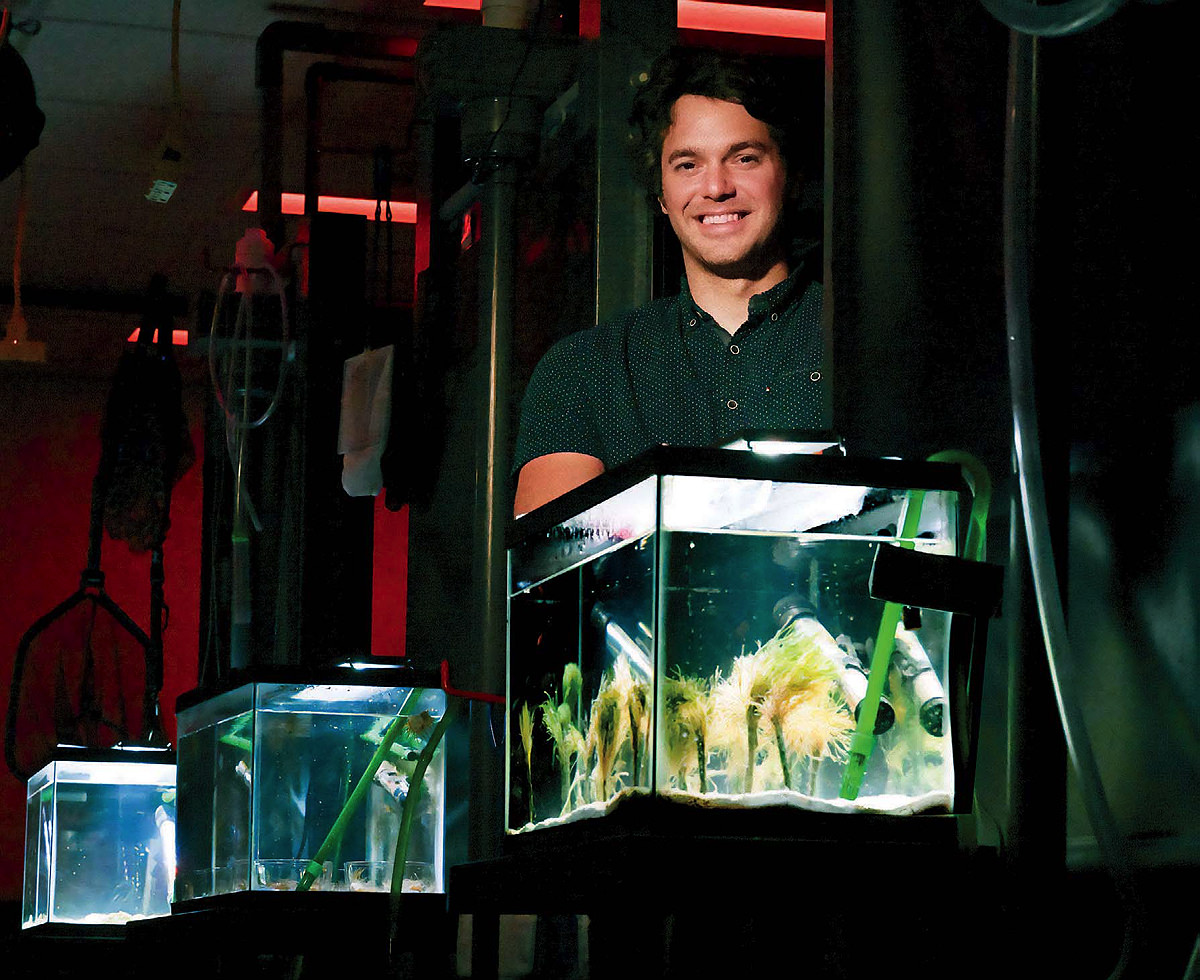
Nicholas Bellono in his laboratory, with sea anemones.
Photograph by Jim Harrison
Since Bellono arrived at Harvard in the summer of 2018 from a postdoctoral fellowship at the University of California, San Francisco (UCSF), his lab has housed an ark-load of animals with marvelous senses and capabilities. A team of three postdoctoral fellows, three graduate students, two staff members (who feed the animals, maintain their habitats, and run behavioral experiments), and a rotating cast of undergraduates study sea robins (fish that walk on leg-like appendages) that can find prey buried in sandy sea bottoms; marine slugs whose backs glow green with photosynthetic plant organelles they’ve somehow assimilated to supplement their energy needs; sharp-toothed piranhas from the Amazon that enter a state of frenzied mass feeding upon detecting an unknown trigger or signal; colorful jellyfish and sea anemones that sting only prey and predators; and, of course, octopuses—those escape artists and masters of camouflage—with sophisticated, distributed nervous systems, three hearts, and long arms to explore their environment. Building the aquariums, pools, pumps, and seawater reservoir to house this menagerie took almost a year and was accomplished with substantial help from building operations managers Gary Goodwin and Chuck Bilikas, now retired. (Bellono plays hockey with them once a week.)
More than a hundred different animal species have cycled through as his team has explored these creatures’ unusual biological traits or behaviors—curiosities, he says, “that even a child can appreciate.” Not all these animals will prove susceptible to Bellono’s scientific approach, which aims to reduce their remarkable biological adaptations to fundamentals: what cells, organelles, and protein products enable these “cool” specializations, he asks, and how did they evolve? The aim of this curiosity-driven basic research is to make “conclusive, or near-conclusive statements,” he says, about the molecular basis for their physiology, development, and behaviors: in scientific terms, their phenotypes.
Visitors to his lab at 16 Divinity Avenue are greeted by a current subject of such investigations: friendly-seeming, big-eyed sea robins that surface in their sandy pool, expecting food. Bellono learned about them from Scott Bennett, manager of research services at the Marine Biological Laboratories at Woods Hole, on a trip to get cuttlefish. “Scott knows I like weird stuff,” says Bellono. “He told me that, anecdotally, these fish can sense buried prey so well that other fish follow them around.” He brought the sea robins back to Cambridge, filled a pool with seawater and sand, and buried mussels to see if the fish could find them. “They were clearly good at it,” he says. But were they sensing the mussels mechanically, by probing the sand? Or did a chemical sensor help them locate prey? To find out, postdoctoral fellow Corey Allard buried capsules in the sand. He filled some with ground-up mussels and the rest with inert materials. When the sea robins found only the capsules with prey, he and his colleagues realized that their leg-like appendages are tipped with chemical receptors. But another New England species of sea robin “couldn’t find anything,” the scientists discovered: they hadn’t evolved this special talent.
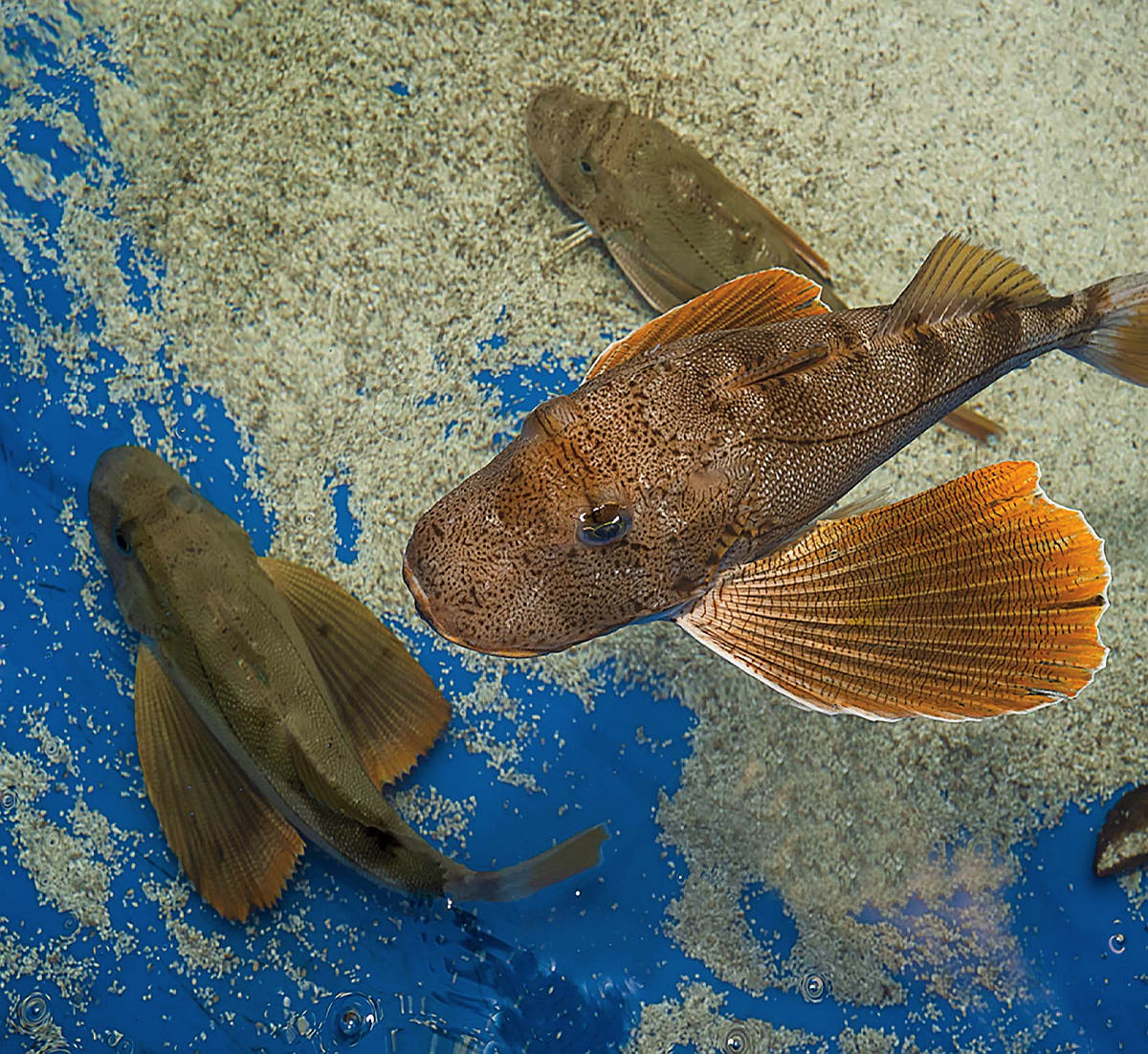
Certain species of sea robins, like these ones from New England, can find objects buried under the sand.
Photograph by Jim Harrison
Next, Allard and Bellono will isolate and study the sensory cells on the sea robins’ appendages to see how they function in their original context. Then they will clone and express them in a cell line, often from a frog egg or a Chinese hamster ovary cell. Because these widely used cell lines are robust and self-sustaining, the researchers will be able to see exactly what protein products the cells’ genes encode outside their native environment, which will help them explore their structure, function, and mode of action.
Stumbling into Science
Bellono was drawn into science almost by accident. He grew up in the Detroit area, where his mother was a social worker and his father worked in the automotive industry. While an undergraduate at Michigan State, he took a job in a lab studying olfaction in salamanders and discovered that he enjoyed working with the animals and, equally, tinkering with the lab’s delicate electrophysiological apparatus used to measure the flow of ions in cells. The work—not completely unlike his father’s, testing car parts or figuring out how to break a system—involved “fabricating tiny glass pipettes that we maneuvered down to make a seal on individual cells,” to measure electrical currents passing through the cell membrane.
“That’s difficult enough,” says Bellono, who later put in “a lot of laborious effort” to apply this technique to the organelles inside cells—another order of difficulty that brought commensurate satisfaction. “I thought that was really fun, that you could be creative and design something,” he recalls. Bellono was particularly impressed by one colleague’s finesse with the apparatus—which requires a steady hand—and with his obsessive approach to the work. “He did it full on, working through the nights….” Bellono thought that was “really cool—that I could go to the lab on a Friday afternoon after classes and just work through as long as I wanted.”
That all-in commitment served Bellono well during his later training, first as a graduate student at Brown (where he studied the pigmentation gene used to map human migration patterns from Africa into Eurasia) and then during his postdoctoral stint at UCSF, when he began studying how sharks detect their prey’s electrical fields. Working in the lab of David Julius, “We didn’t have tanks,” he explains, so “we’d get the sharks sent from Woods Hole, do the dissection, put the cells on ice, and then stay up for three days doing experiments.” He would keep doing that until he “could say something about how the native cells worked.” It was during this period that he acquired the skills necessary to take an interesting phenotypic problem and reduce it to one molecule: a single protein. He learned this approach—how to identify and clone a protein based on function—from Julius, who “has done it numerous times, very, very well,” including to probe the molecular basis for pain sensation (for which Julius was awarded the Nobel Prize in Physiology or Medicine in 2021).
From Function to Fundamentals
Bellono has applied this method to understand a variety of intriguing animal behaviors and adaptations and their underlying structural and molecular mechanisms. First, he explored the electrosensory organs of two types of ancient cartilaginous fish—sharks and skates—which can detect the weak electrical fields of other marine animals. Sharks use this ability to hunt prey, while skates use it mainly for communication with other skates. Bellono wanted to know if these behavioral differences might be reflected in structural differences between the cells the sharks and skates use to detect electrical fields. Had their electroreceptor cells evolved differently?
What he found, as described in a paper that became a 2018 Nature cover story, were “subtle molecular variations [that] considerably alter cellular properties”—enough to lead to differences in behavior. In sharks, he discovered, the molecular structure of the electroreceptors supports amplified, indiscriminate signal detection. That enables sharks to detect any living thing—perfect for an omnivorous apex predator using this specialized sense to find prey.
But in skates he found cellular receptors that are optimized for detection of selective electric-field frequencies. Unlike sharks, Bellono explains, skates can both detect and generate electrical fields. The frequencies skates generate (from an organ at the base of their tails) change, “so if they’re in mating season, they produce a different frequency” than they do at other times. “And in the wild, if you play back a recording” characteristic of the reproductive season, “they’ll respond if they are in mating condition.” Electroreceptors that are enhanced for receiving these particular signals would aid in skates’ reproductive success. Bellono wonders if this seasonally shifting receptivity is accompanied by structural changes in skate electroreceptors during the breeding season.
“I don’t know if it is true, and we were never able to ask whether the skate sensory cell actually changes its properties in different seasons. Does the frequency sensitivity shift?” It’s a question he still hopes to explore, “something that I always wanted to do here and have never been able to because everybody’s working on a zillion different animals. It’s great to be in the business of exploration,” he explains, “but sometimes it comes at a cost where we don’t get to explore deeper some of the things that I wish we would.”
Octopuses are the exception. Bellono thinks that maybe when these creatures explore with their arms, they are not chemically sampling the objects they touch, but rather the community of microbes that grow on their surfaces. This might be what enables octopuses to distinguish the shell of a crab—their favorite food—from a rock, for example. The chemical detectors in octopus receptor cells are comprised of five subunits, which can be combined in different molecular-level configurations to recognize different chemicals. Bellono wonders if this equips the octopus to sense a greater range of things: could one combination detect a crab and another detect something else? In 2017, a different lab discovered that octopuses can edit their own RNA and thereby change the proteins they make. Bellono wonders whether that capability exists to facilitate shifts in their chemotactile receptors, enabling them to encode slight structural differences in order to sense what they want in their seafloor environment.
Propelled by Fascination
During their continuing studies into this question, Bellono’s team, together with assistant professor of cell biology Amy Lee, recently made a strange discovery: “The octopus has a structurally distinct ribosome”—the intracellular organelle that synthesizes proteins—“compared to all other animals. It is very weird, but it seems to alter the accuracy by which the octopus makes proteins, so that it very rarely makes mistakes, as compared to its shelled mollusk relatives.” The scientists are only beginning to explore the biological implications. “I have no idea really what it means,” he admits, “but it may apply very broadly biologically to the question of how organisms generate new traits—to me, among the most interesting questions.”
“I encourage students…to explore everything with the kind of wonder that we felt about the world as kids, because that’s where a lot of discovery comes from.”
It’s also an example of how Bellono’s research proceeds, by remaining open to new questions and discoveries, “so that we can stumble into something that is interesting.” For him, scientific inquiry is both a creative process and an art—something he tries to teach his students. “I encourage them to be curious and open-minded, to explore everything with the kind of wonder that we felt about the world as kids,” he says, “because that’s where a lot of discovery comes from.”
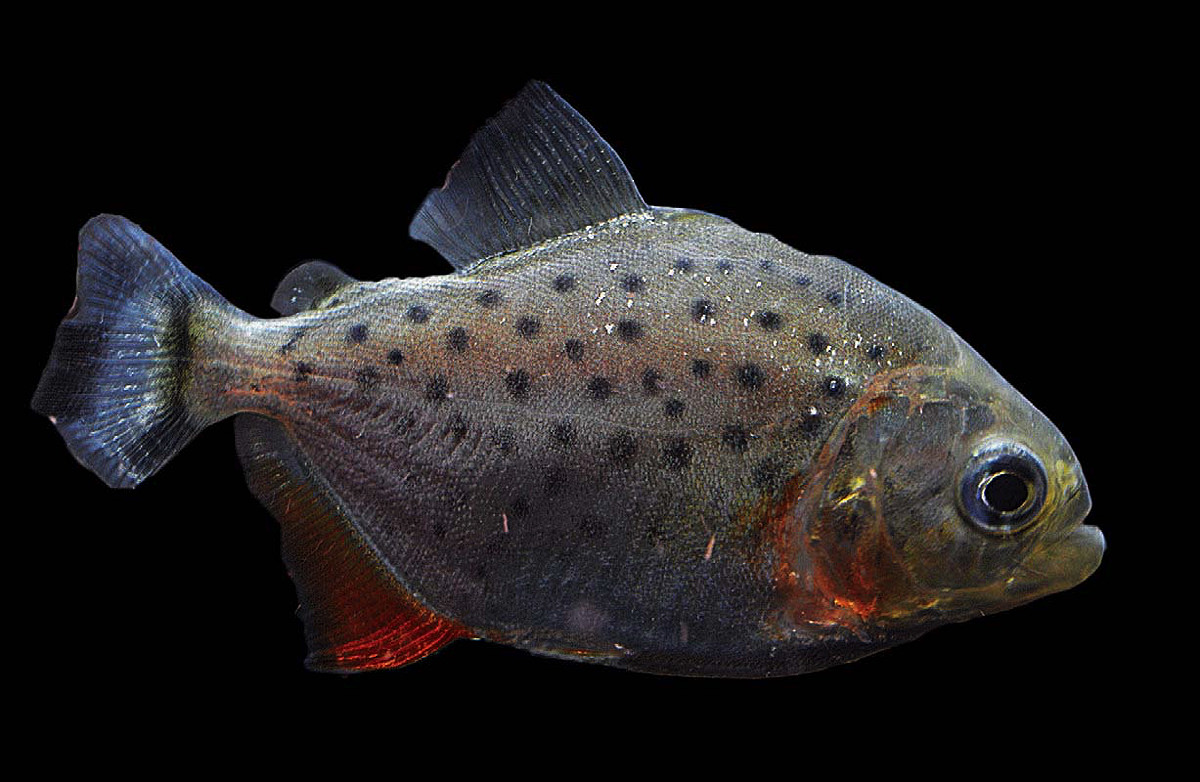
Piranhas are well-known for their frenzied feeding behavior. But what cue triggers this group behavior, and how is the signal transmitted or received?
Photograph by Anik Grearson/Bellono Laboratory
This is the credo that brought pirhanas to Bellono’s lab. As research assistant Peter Kilian feeds them capelin, a fish in the smelt family that the piranhas love, he explains how he saw a television show in which someone poured blood into a pool of piranhas and the fish went crazy. “Then he got in the pool,” recalls Kilian, “and the fish ignored him.” Then, when he threw in a piece of meat, they went into another feeding frenzy. “How do they know the difference?” Kilian wondered. After securing the necessary permits (piranhas are illegal in Massachusetts), the lab has recently begun trying to figure out what the fish might be sensing.
Although Bellono’s research to date has focused primarily on aquatic animals, his molecular approach could apply to terrestrial systems, exploring such species in precise ways they’ve not been studied before. Accordingly, he is equipping a lab room to house reptiles and amphibians, which “have all kinds of crazy adaptations. There’s a huge diversity,” he notes. One student recently joined the lab to figure out how tree frogs can hatch prematurely from their eggs when a predator nears, somehow sensing the vibrations of a slithering snake. Bellono himself is fascinated by a wood frog that can completely freeze in the winter—the frog “turns into a block of ice—and then is still alive afterward,” he says. “It’s pretty nuts.”
Nor does that end the oddities Bellono intends to explore scientifically. He also plans to study metamorphosis in amphibians, both because a pollywog becomes a whole new animal, but also because it transitions from being aquatic to terrestrial, which requires the development of a whole new set of organ systems in a very short time. “We’ll be able to study something that changes, not as a product of evolution over many years,” Bellono anticipates, “but in a matter of weeks.”