The most versatile chemists in the world live inside us. Trillions of microbes, of several hundred species, an aggregation containing 150 times as many unique genes as a human, make our bodies their home. More biological activity may take place between and among these helpful hitchhikers than in our own cells. Their astonishing abundance and genetic diversity are matched by a vast repertoire of products and functions—inputs, outputs, and biochemical interactions—that profoundly affect human health.
Many microbes are beneficial, breaking down foods, manufacturing vitamins, and offering protection against pathogens—including, scientists recently discovered, viruses. But some increase the risk of cancer, cardiovascular disease, and diabetes, while others have been linked to neurological diseases such as Parkinson’s. A few are even known to metabolize drugs, blunting the effects of therapies administered to help maintain our human health. But given the scale and complexity of this vast sea of microscopic life, discerning which microbe is responsible for any of these effects—separating the good guys from the bad—has proven extraordinarily challenging.
A human chemist, Emily Balskus, Ph.D. ’08, is starting to address that problem using inventive strategies inspired by her training in organic chemistry. She studies the microbiomes of the human gut and recently, the vagina, by characterizing the metabolic activities taking place in communities of microbes that are associated with health on the one hand, and with disease on the other.
Broadly speaking, she says, microbial diversity is a good thing in the gastrointestinal tract. There, lots of microbes interact with their hosts, the foods they ingest, and with each other, forming metabolic chains and networks: one microbe’s trash is another’s treasure. (The production of many foods, of course, depends on domesticated microbes: yogurt, cheese, bread, and wine are just a few.) In the vagina, by contrast, such diversity is disadvantageous. The healthy vaginal microbiome is dominated by a single genus of bacteria, Lactobacillus, that maintains a protective, slightly acidic environment. If certain other microbial species become established in that ecosystem, unhealthy outcomes may follow: increased risk of preterm birth, vaginosis, and HIV infection.
Beyond these general findings, there is clearly much more to learn about the human health effects of the microbiome, and about how to intervene safely when things go awry. Yet studies of microbial communities have been limited: genetic sequencing technology reveals which organisms are present, but those DNA readouts don’t tell scientists what activities are linked to particular species or genes. And standard methods for discerning the identities of each microbial species and the products of their metabolism—their life-sustaining chemical reactions—often don’t work: many microbes have never been successfully cultured in a petri dish, or in any other way.They are known to science through their DNA. The fact that there are a billion microbes in a gram of soil, and a billion in a liter of sea water, hints at the scale of this problem. The human colon, the final stretch of the gastrointestinal tract, is at the apex in terms of microbial abundance and complexity: it’s among the most densely colonized places on the planet.
Figuring out what all those microbes do has become the central challenge of Balskus’s career—one she hopes she and other microbiologists can begin to solve within the next decade.
A woman in a man’s field
A young professor of chemistry and chemical biology with a list of awards and honors that already stretches onto a second page of her C.V., Balskus is the rare woman in her field. The eldest of four children, she was born in Columbus, Ohio, and raised in Cincinnati, where both parents were teachers. “Education was part of our lives,” she says, “and the rhythms of the school year were part of the fabric of our family.” The steadiness of this academically supportive environment, and her natural joy in learning new things, now define her pioneering approaches to tackling problems in microbial science.
Nevertheless, throughout grade school, Balskus felt that she didn’t fit in, socially or intellectually. That changed when she attended Ursuline Academy, an all-girls Catholic high school with an outstanding science curriculum. “Being in a culture of excellence where women did everything—leading student government, succeeding as athletes, excelling academically—was very helpful in building my self-confidence,” she says. One teacher in particular, Diane Rose, catalyzed her passion for chemistry. Balskus recalls the day during her sophomore year when she arrived in Rose’s class unprepared, having skimmed the required reading. Asked to answer a question about the homework, she faltered, but managed an adequate response. Rose asked to see her after class.
“I’m in trouble,” Balskus remembers thinking. But instead of a reprimand, Rose challenged her. She asked whether the class was moving too slowly for Balskus, and offered to supplement the standard curriculum with extra topics and additional instruction. “That made a huge impression on me,” Balskus says now. To have somebody recognize her aptitude and potential “meant a lot.” And she began to wonder what she might achieve if she invested more time and effort. As she did so, chemistry became increasingly “fascinating and fun.”
Still, it was a man’s field. During her sophomore year at Williams College, in 2000, two professors took her aside at different times and suggested she think “really hard” before going into organic chemistry, because it was a field with very few women. Balskus could name just one female organic chemist at a top university at that time, the year 2000. “And at Harvard,” she says, “there were no women on the organic chemistry faculty, and research groups were known for being male-dominated and really rough places. But I loved the science.”
The following summer, she went to work for a well-known chemist, Leo Paquette, at Ohio State. He had never had a female undergraduate in his lab. As “the very first one, I came in with my eyes wide open and feeling a little uncertain about it. But he was an amazing mentor”—as was the Puerto Rican graduate student she worked with. “As a member of a different underrepresented group,” she says, “he certainly faced his own obstacles to making his way in organic chemistry.” But on “both a scientific and personal level, he really made me feel that I belonged in that lab, and in that field.”
After college, Balskus spent a year earning a master’s at Cambridge University, before entering a doctoral program at Harvard in the lab of organic chemist Eric Jacobsen, an expert in controlling catalytic reactions. She specialized in a type of chemical reaction that takes place so fast that some people didn’t think catalysts could be used to control them. But Balskus succeeded, and then used the reaction she’d developed to replicate a natural product made by sponges. Her way of building the molecule was different from nature’s way. The product itself may have been unimportant, but her work was valuable as a proof of concept: it showed that chemists could develop efficient and elegant ways to build such molecules. Many of these lab-produced “natural products” are used as drugs (salicylic acid, for example, originally used to make aspirin), or inspire the development of pharmaceuticals. For Balskus, who aims to design therapies for treating the human microbiome, this new skill acquired in chemical synthesis proved to be only the first of many.
During her graduate studies, she also began running—and decided to tackle the Boston Marathon. Jacobsen was supportive: training for the marathon would translate well to science, he told her, because it requires overcoming obstacles and working toward long-term goals—sometimes through pain and discomfort. Balskus found it “fun, fulfilling, and a great way to relieve stress.”
Her turn from organic chemistry to microbiology took place during a postdoctoral fellowship with professor of biological chemistry and molecular pharmacology Christopher T. Walsh (now emeritus). In his lab at Harvard Medical School, Balskus became fascinated by bacterial physiology. The project she and Walsh devised was a study of cyanobacteria—blue-green algae. These primitive plant precursors are credited with oxygenating ancient Earth’s atmosphere, which eventually enabled the flowering of life on the planet. “Cyanobacteria need sunlight to survive, but face the challenge of exposure to damaging ultraviolet [UV] radiation,” Balskus explains. Over time, they have evolved ways to synthesize natural sunscreens—her initial research focus. “You can imagine that in order to absorb UV, and not do damage to the cell,” she explains, “they have to have some pretty interesting chemical structures.”
Her interest in the protective sunscreens evolved into a fascination with the biosynthetic pathways that produced these chemical structures, and with the enzymes that catalyzed the key reactions. Enzymes are nature’s catalysts: biological molecules that accelerate biochemical reactions in living organisms. Balskus began reading about the ecology of cyanobacteria, and their adaptations to even the most inhospitable environments.
Reading the microbiome
“As I developed a greater appreciation for how hard it is for synthetic chemists to make molecules in the lab,” she says, “I became interested in how nature made molecules. And then I was drawn to microbes, because they make some of the most beautiful natural products, using these beautiful protein-based catalysts, that had been so hard for me to make in the lab. As a chemist, I wanted to know how they did it.”
Walsh recognized her burgeoning interest in microbiology before she did, she says, and encouraged her to spend a summer at the Marine Biological Laboratory in Woods Hole, on Cape Cod. There she got a crash course in microbial diversity, learning to culture the relatively few microbes (bacteria, archaea, fungi, protists, and viruses) that have been cultured to date, and to sequence the DNA of the rest.
“Microbes…make some of the most beautiful natural products…. As a chemist, I wanted to know how they did it.”
And it was there that Balskus came face to face with what she calls “the next big problem in biology”: the functional characterization problem—how can a microbe be linked to its metabolic activities? At the first few scholarly microbiome conferences she attended as a professor (she was appointed assistant professor of chemistry and chemical biology in 2011), Balskus was the only person who wasn’t talking about sequencing microbes. Instead, she spoke about discovering enzymes, emphasizing that scientists don’t know what a lot of the genetic material they had begun sequencing actually does.
The problem is enormous, given that “85 percent of the genes in the healthy human gut microbiome can’t be confidently linked to a function,” Balskus says. “And 70 percent of the microbial diversity in the gut hasn’t yet been cultured,” preventing scientists from isolating, growing, and studying the microbes themselves in a petri dish.
Disentangling one microbe’s genes and activities from another’s within a genetic soup is made all the more challenging by the mutable nature of those activities. Some strains of E. coli, for example, are harmless, while others can make people extremely ill. Balskus notes that the genes can differ by more than 50 percent among these strains, but the strains themselves are still considered part of one species because of similarities in certain core genes. Those differences come about in part because bacteria are adept at swapping entire groups of genes that together encode a specific metabolic function. Even ostensibly unrelated bacteria can share these common pathways through a process called “horizontal gene transfer” (a lateral swapping of genetic information that does not require reproduction).
Given this complexity, Balskus realized that in order to study functions in the microbiome, it was important to link those functions to the genes that encode them, rather than link them only to particular microbial species. Knowing the identity of the organism producing a specific molecule might not be necessary, she explains. It could even cause confusion: there might be more than one source of a particular molecule within a gut microbiome if different microbes share similar capabilities. From her point of view as a chemist, what matters is the product and the pathway that makes it—not the microbe itself. “We need to develop methods for directly detecting functions of interest,” she says.
Bringing a chemist’s perspective to that problem, she has focused on characterizing the molecular reactions at work. She realized that her goal of using enzymes (biological catalysts) to control metabolic reactions within microbial communities—to develop therapies, in other words—would work no matter the specific source of any particular metabolic product. Those metabolites could be beneficial, such as glucose, vitamins, or antioxidants, or harmful, like the free radicals that can damage DNA, so long as the chemical mechanisms could be deduced.
Chemical detectives
One early example of the sophisticated biochemical sleuthing performed in her lab involved the metabolism of choline, a component of the lipids that make up cell membranes throughout the body. Humans produce choline, but not enough to maintain optimal health, so they need to supplement the supply through their diet, with foods such as egg yolks, dairy products, and certain fruits and vegetables. But some people’s microbes break down choline into harmful byproducts, one of which is converted in the liver into a compound linked to cardiovascular disease, diabetes, and diseases of the liver and kidneys. Balskus identified a key step in the chemical transformation of choline that she guessed might be similar to related biochemistry already occurring naturally in well-studied bacteria. Working backward with this hypothesis in mind enabled her research group to identify a previously unknown cluster of genes in the human microbiome that metabolize choline, and ultimately to zero in on an enzyme responsible for producing the harmful metabolite. Targeting that enzyme, even without knowing its source, could lead to treatments that prevent the harms of microbial choline metabolism.
Recently, her lab discovered genes and enzymes in the human gut microbiome that metabolize cholesterol and that could have a healthful, cholesterol-lowering effect. The byproducts of such metabolism had previously been detected in humans, but the microbes and methods responsible remained a mystery. After much searching, graduate student Doug Kenny decided to study a cholesterol-metabolizing microbe he could culture from the pig-gut microbiome. This allowed him, together with Balskus and Isselbacher professor of medicine in the field of gastroenterology Ramnik Xavier (co-director of the Broad Institute’s infectious disease and microbiome program) to analyze the biochemical transformations at work—and then to search for similar chemistry in humans.
Cholesterol metabolism in the human microbiome turned out to involve a different type of bacteria. Individuals who possess the cholesterol-metabolizing organisms (which are among those that have never been successfully cultured) have lower levels of cholesterol in both their stool and their blood. “If we could figure out how to increase this activity” or introduce it into the many people who lack this pathway, Balskus says, “it could potentially have a huge impact on treatment of heart disease.” There is more than one way to achieve this, she notes. “One would be to engineer an organism to consume cholesterol.” Another would involve stimulating the growth of these organisms naturally, by figuring what dietary factors might fuel their growth.
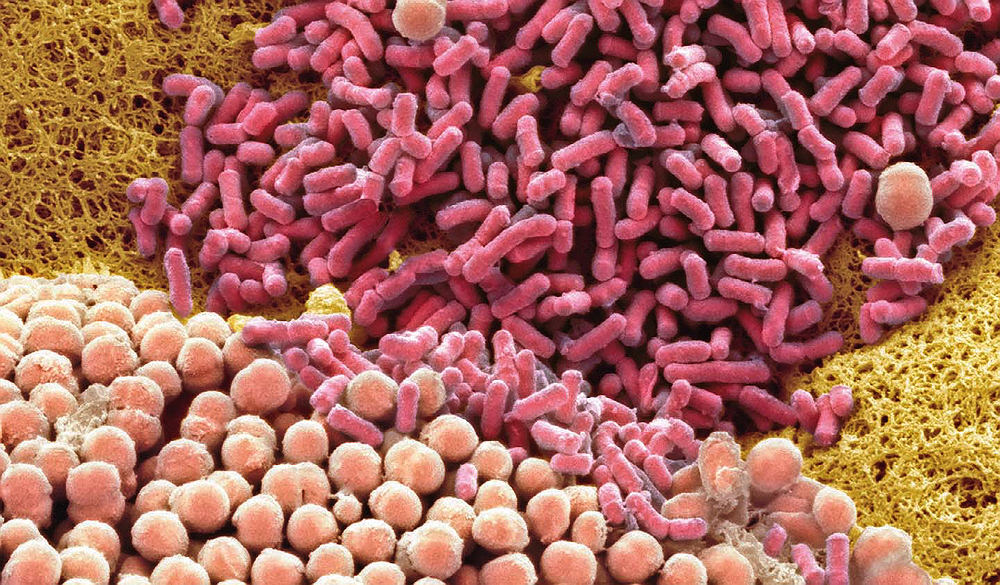
This colored scanning electron micrograph shows cultured cervical bacteria. Balskus is part of a multidisciplinary team studying the vaginal microbiome’s impact on women’s health.
Image by Steve G. Schmeissner/Science Source
Excited by the potential for this health-promoting type of research, Balskus is carrying that enthusiasm to a new, Gates Foundation-funded project focused exclusively on women’s health. The consortium of researchers seeks to identify the hallmarks of stability and resilience in vaginal microbiota, aiming to move from basic scientific discovery toward development of an intervention to help prevent preterm birth and HIV infection. The Gates Foundation consortium includes research and clinical immunologists, experts in modeling complex biological systems, and scientists who can sequence the vaginal microbiome’s genes. Balskus’s role is to figure out what enzymes enable health-promoting Lactobacillus strains to thrive, and to discover which organisms are associated with imbalances in natural microbiota. “We often don’t know the enzymes responsible, but if we did, that might help us select strains that could be used in a probiotic” to promote beneficial microorganisms, she says. “It could also help us understand how to manipulate that environment to prevent the growth of potentially harmful organisms without using antibiotics, which don’t work well for many patients.”
. . .
In the decade ahead, Balskus hopes to integrate more of the chemical knowledge that she teaches her students with both the reams of big data streaming from clinical research, and with large-scale biochemical screening of gut microbes that has revealed hundreds of metabolic events that “we didn’t know were happening.” Microbiome-targeted therapeutics—whether in the form of small molecules (drugs), organisms themselves, food for microbes, or probiotics—are all progressing toward clinical application, and researchers will soon learn, she hopes, which of them work—and why. “That mechanistic information,” she says, “will be really useful, I think, in deciding how we move forward in developing therapeutics that target these organisms.”
“I’m drawn to these new projects,” Balskus continues, “often because they give me the opportunity to learn something new and to explore different fields. And that’s one thing I really like about being able to work in the human microbiome—that we get to learn about human biology. That’s something very new to my lab, but it has provided a lot of interesting opportunities to continue to grow and to expand my scientific knowledge.”