As a young man, Sharif Tabebordbar remembers seeing his father struggling to play soccer, and then losing the ability to ride a bike. He could only watch as his father declined, his once healthy body ravaged by a degenerative muscle disease that ultimately confined him to a wheelchair. He decided then that he would try to find a cure for his father’s illness. Now, an interdisciplinary team of Harvard students assembled by Tabebordbar and based in the lab of Pardis Sabeti at the Broad Institute of Harvard and MIT, together with help from stem cell scientist Amy Wagers, and other Harvard-affiliated labs, has overcome a key stumbling block that has stymied previous efforts to cure genetic diseases using gene therapy. The team has developed a way to deliver gene repairs that specifically target muscle tissue, and that could potentially be used to target other tissues as well. Their findings were published online September 9 in Cell.
Gene therapy, decades ago, got off to a troubled start. The earliest clinical efforts to deliver repaired genes used adenoviruses as delivery mechanisms to disseminate the instructions for repairing defective genes. But adenoviruses are immunogenic—they stimulate an immune response. This makes them ideal for delivery of vaccines (such as the Astra Zeneca and Johnson & Johnson COVID-19 vaccines) but not for gene therapy, in which the aim is to quietly deliver a genetic fix to mutated genes that cause disease without stimulating an immune response. Researchers in the field have therefore focused instead on using naturally occurring Adeno-Associated Viruses (AAVs), a class of harmless viruses that are endemic in humans, to deliver gene repairs in vivo.
The problem with this approach has been that AAVs have an affinity for the liver. When injected systemically, as much as 90 percent of the curative viral payload ends up in the liver, rather than in muscle, or other tissues targeted for repair. In pre-clinical and clinical trials, many gene therapies have been shown to work: they effect the repairs for which they were designed. But because most of the therapeutic viral vector ends up in the liver, the cures must be administered in massive doses in order to ensure that sufficient quantities reach the desired target—muscle tissue. And those massive doses can be dangerous because they reach levels that are potentially toxic.
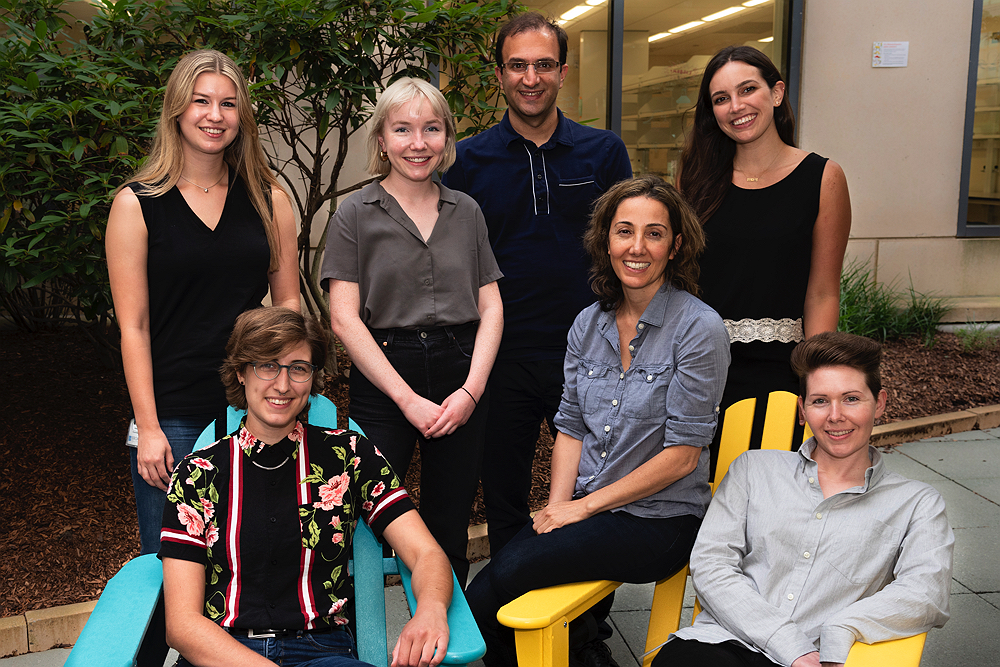
Standing, left to right: Allison Krunnfusz, Emily King, Sharif Tabebordbar and Liana Tellez. Seated: Allie Stanton, Pardis Sabeti, and Kim Lagerborg.
Photograph courtesy of the Broad Institute/Allison Dougherty
To overcome this problem, Tabebordbar, an associate of the department of organismic and evolutionary biology, and his colleagues used directed evolution to grow and select for AAVs with an affinity for muscle, but not other organs and tissues. They selected a strain that showed some activity in muscle tissue in mice, and then created 30,000 more related AAVs, using the expertise of computational biologist Simon Ye, (a doctoral candidate in the Harvard MIT Health Sciences and Technology program who previously worked on the Google Glass project), to sift through the resulting variants and find the ones with the greatest affinity for muscle. And then they started the process again, says Ye, “using only the variants that showed a strong result.” Screening for the best candidates at such a massive scale is enabled by “relatively modern technology,” he continues. “Fifteen years go, people were cloning viruses by hand,” so they could only choose one or two viruses to use as the basis for creating a new one, “whereas we are testing more than 100,000. It’s a huge advance in our capabilities.”
After many generations of selection, the team created an AAV with such strong affinity for muscle that it remains effective even with 100 to 150 times less virus than other viral delivery vectors. Although developed first in mice, MyoAAV (a family of viruses so-called because they home in on muscle fibers called myotubes), was also tested and evolved to work in non-human primates in vivo, as well as in cultured human cells in the lab.
The entire process required enormous amounts of bench work. While Tabebordbar led the research strategy, co-lead author Kim Lagerborg, a doctoral candidate in Harvard’s Biological and Biomedical Sciences (BBS) program, coordinated most of the experimental efforts. “I enjoyed training and mentoring others” she says, as she kept the research on track and on schedule. That’s no easy task when “every single tissue from every single experiment must be processed individually to make sure that we find the variants that are most potent in the skeletal muscle, but nowhere else. I’m amazed when I step back and look at the paper, to see how all these little experiments can turn into one tiny graph.”
Allie Stanton, a member of the Sabeti lab pursuing her Ph.D. in the Harvard program in virology, was another key contributor who conducted many of the wet-lab type experiments in cells, drawing on her background in molecular biology, which she studied as an undergraduate at MIT. She performed much of the work on the receptor that MyoAAV latches on to in muscle.
In interviews, both she and Lagerborg cited the potential for helping cure disease as a reason for signing on to the project. “Always in your head, there’s this possibility that what you are working on could really reduce the amount of suffering that someone is experiencing,” said Stanton. “I think we’ve made some real progress. I’m hopeful that someday, somebody will be able to see an improvement in their life because of what we have done here.”
Tabebordbar, of course, was the driving force behind the project. After receiving bachelor’s and master’s degrees in biotechnology from the University of Tehran, he earned a Ph.D. at Harvard in 2016 in the lab of Forst family professor of stem cell and regenerative biology Amy Wagers, where he developed a method for correcting the genetic mutation that causes Duchenne Muscular Dystrophy. That work was published in 2016. But he approached professor of immunology and infectious diseases and professor of organismic and evolutionary biology Sabeti, a virologist, when he discovered that the major breakthrough needed in gene delivery was dependent on the ability to evolve and engineer viruses. The collective student expertise in Sabeti’s lab—in virology, computational biology, and biological and biomedical science—was perfect for what Tabebordbar was trying to accomplish, says Sabeti. “He had a very ambitious program that required an interdisciplinary approach, but rooted in viral evolution. As a faculty member, the pure delight of this kind of work, and the goal, is to bring brilliant people together on a common mission and let teams shine. This work exemplifies that spirit.”
Although Tabebordbar has been focused on curing degenerative muscle diseases, the system that he and his team developed for evolving AAVs is perhaps the most significant contribution of their work because it could be adapted to exclusively target other tissues and organ systems, potentially accelerating the clinical application of gene therapies and other forms of genomic medicine to fight a range of human diseases.