Pellegrino University Professor emeritus E.O. (Edward Osborne) Wilson has been acclaimed for work across an astonishing range of life sciences: study of individual ant genera; explicating biodiversity and sociobiology; and, of late, increasingly urgent warnings about the fragile, endangered web of species worldwide. Harvard Magazine has profiled Wilson (“Of Ants and Earth,” March-April 2003), and excerpted his memoir, Naturalist (“The Molecular Wars,” May-June 1995).
Now he is the subject of SCIENTIST: E. O. Wilson: A Life in Nature, by Richard Rhodes, a Pulitzer Prize winner (an honor Wilson, a superb writer, has himself won twice). The following excerpt, from chapter 5, shows Wilson making the transition from his early work on classifying ant species to understanding their behavior through molecular science. During this time, Wilson found himself at odds with James D. Watson, the co-discoverer of the structure of DNA (for which he later shared the Nobel Prize); both were young, ambitious, rising faculty members at Harvard, at a moment of fundamental change in life-sciences research. Wilson described their collision in the excerpt from Naturalist, where he characterized Watson as a “brilliant enemy” and an “adverse hero.” Here, Rhodes focuses on Wilson’s inherently interesting subject of pheromones and ant communication, his experiments and discoveries, the limits of what science could accomplish given the apparatus and techniques available then, and the foundation he and his colleagues established for the advances to follow. ~The Editors
* * * *
Digging into an ant colony in the field sends workers and queens scattering everywhere. That was no way to observe them, much less to experiment with them. To experiment with ants, Ed Wilson worked out a way to keep them in the laboratory. Since his undergraduate days in Alabama, he had improvised various kinds of tubs and boxes for ant keeping. By the time he moved on at Harvard from collecting and classifying to doing experimental work, in the late 1950s, he had developed a dependable laboratory ant habitat: a multilevel Plexiglas box about three feet long and two feet high. A sliding wall allowed access for cleaning or manipulation. The floor between the levels was made of softwood strips; a curved plastic tube extended through the wall to allow the nest to be watered. A single door in the bottom gallery of this artificial nest opened to a walled foraging area—an outdoor pen, as it were—where the colony could be observed as it displayed its behaviors. The nest interior was brightly lit, light to which the ants had been gradually habituated. Fluorescents illuminated the foraging area but operated on an automatic twelve-hour timer, simulating the cycle of day and night. The entire habitat could be covered with a Plexiglas lid, but Wilson normally left it open for easy observation and handling. A coating around the rim, of a commercial fluoropolymer resin, Fluon, which dried too slippery for the ants to cross, kept them from escaping.
“If I could find this part of the ant Rosetta Stone,” Wilson wrote, “I could ‘speak’ to a fire-ant colony and tell them where to go for food.”
The Beer Can Technique
Wilson’s preferred study subjects were fire ants, despite their small size, about one-sixteenth of an inch, and their painful stings. They were plentiful and easily acquired. On weeklong excursions, Wilson would drive to South Carolina or Alabama, alone or with a graduate student or two, park on a rural roadside beside a fire-ant mound—they were everywhere now in the South—fetch a garden spade and a bucket from the trunk of his car, deposit a flattened beer can nearby, then dig the spade deep into the mound and scatter a spray of dirt and agitated ants along the roadside. Inevitably, he’d suffer stings, but a few fire-ant stings were a small price to pay for a living colony to transfer to his habitat in the Harvard Biological Laboratories. In the mêlée of the scattered colony, the ant queen’s attendants would spot the flattened beer can and hustle her over to hide her underneath. All Wilson had to do then was to turn over the beer can, catch the queen with forceps—she was many times larger than her crumb-sized workers—slip her into a collecting tube, and pop on the lid. He called the method the Beer Can Technique. A few scoops of workers dropped into the bucket along with the queen in her tube, and some paper toweling for shelter, and he had a functioning colony to transplant to the laboratory. If a highway patrolman came along and stopped to find out what he was doing, he told the officer he was working for the government. That was all it took, and he was, at least technically, since his research grants came from the National Science Foundation.
Back at Harvard, Wilson prepared a nest box and moved the colony in. The queen would soon enlarge it, laying eggs at such a rate that it would have reached 20,000 workers or more within a year had Wilson not kept it culled. He maintained his research colonies at about 100 individuals, more than enough for his studies, and easier to feed and maintain.
That ants communicate by smell had been known since at least the late nineteenth century. The Swiss neuropsychiatrist Auguste Forel, an amateur myrmecologist whose major study of the ants of Switzerland had won praise from Charles Darwin, summarized the importance of smell in ant life in his 1908 book, The Senses of Insects:
It can therefore be boldly supposed that the antennae [of ants] and their olfaction [i.e., their sense of smell], as much on contact as at a distance, constitute the social sense of ants, the sense which allows them to recognize one another, to tend their larvae and mutually help one another, and also the sense which awakens their greedy appetites, their violent hatred for every being foreign to the colony, the sense which principally guides them . . . in the long and patient travels which they have to undertake, which makes them find their way back, find their plant-lice and all their other means of subsistence.
But what were they smelling? What secretion or secretions could determine in an instant if one ant welcomed another ant into the nest or furiously attacked it? Bees that discover a field of flowers returned to the hive and performed a waggle dance to teach their sisters where to find the new source of food; ants, operating in two dimensions and often in darkness, apparently laid down a scent trail to recruit their kin. And the actions of ants in response to such chemical markers were compulsory, not voluntary.
Organic compounds that are secreted inside the body to regulate some specific cellular activity are called hormones. In 1959, in the journal Nature, two European zoologists proposed a new name for the analogous compounds then being investigated that are secreted outside the body for communication between individuals: “pheromones.” In their new coinage, the Greek word from which “hormone” is derived, ormoni, meaning “to excite,” supplied an ending to another Greek word, pherein, meaning “to transfer.” “Pheromones,” the zoologists wrote, “are defined as substances which are secreted to the outside by an individual and received by a second individual of the same species, in which they release a specific reaction, for example, a definite behavior or a developmental process.”
“Humans communicate by sight and sounds,” Wilson comments, “allowing the creation of words with arbitrarily chosen meaning. In other words, we communicate by language, the prerequisite for the most rapid possible evolution of social order. Ants, in contrast, use chemical secretions, smelled or tasted, with gene-fixed meanings.”
The “Ant Rosetta Stone”
But no one had yet identified those chemical secretions, nor had their source within the ant’s body been found. In the summer of 1958, Wilson decided to try to find and identify them. He had already realized that the trail ants lay down to guide their fellow workers to a newly discovered food source must be a pheromone. He had decided as well that the best way to determine which gland or glands produce the trail pheromone was to test extracts of various glands by laying artificial trails with them and observing how his laboratory ants responded. “If I could find this part of the ant Rosetta Stone,” he writes, “I could ‘speak’ to a fire-ant colony and tell them where to go for food.”
So he would be looking for an exocrine gland—one that discharges its secretion through a duct to the outside of the body, as human tear and saliva glands do. That limitation pointed him to the fire ant’s mouth at one end or stinger at the other, its body’s obvious openings to the outside world. He had already noticed that fire ants, laying trails, curled their gasters—the bulbous rearmost segment of their bodies—under them and touched their stingers intermittently to the ground, “much like a moving pen dispensing ink,” which directed him to the gaster as the likeliest place to search.
The body of even a small ant is packed with organs and glands. Fire ants are among the smallest, their internal parts diminutive. Wilson had neither the time nor the money that summer to buy and learn to operate a complex micromanipulator system in his laboratory. Instead, he relied on a large magnifying glass mounted on a bench stand, his one, exceptional eye, and what he calls “the finest of all handheld instruments, the needle-tipped Dumont Number 5 forceps, used by jewelers to handle very small precious stones.” Even then, the scale at which he was working ruled out deliberate movement. When he tried deliberate dissection, overcompensation scattered ant parts everywhere. He soon learned to take advantage of the hand’s normal tremor, its fine, hammerlike response to the pulsing of the blood. “These involuntary movements were just enough to cut through the base of the glands,” he recalls, “and ease them into insect-blood saline for further preparation.”
Since fire ants appeared to mark a trail with their stingers, Wilson looked first at the fire-ant poison gland. “The pheromone might prove to be the venom itself,” he theorized. “But when I tested this hypothesis, the result was wholly negative. Venom meant nothing to the hungry fire ants. Nor did, less surprisingly, the contents of glands I tested from other locations throughout the body.”
Then he noticed another possible source, a small, sausage-shaped gland that opened into the base of the stinger: Dufour’s gland, named for the nineteenth-century French physician and naturalist Léon Jean Marie Dufour, who first described it in 1841. “Barely visible to the naked eye as no more than a tiny white speck,” Wilson writes, “it seemed an unlikely candidate for so important a role as the fire ant odor trail.” He teased one loose from the opened gaster of a freshly killed ant, washed it in saline, and crushed it against the tip of a sharpened applicator stick. With this unlikely brush, he painted a trail from his fire-ant colony’s nest entrance out to the middle of its foraging area. “The result was stunning,” he reports. “The ants in the nest didn’t just follow the trail in modest numbers, they exploded out in a crowded column. They streamed back and forth and all along the trail I had laid. They were like people reacting to a very loud fire alarm in a crowded building, running out and about and shouting.” As if making first contact with an alien life-form, Wilson had spoken to his ants, and they had heard him and responded.
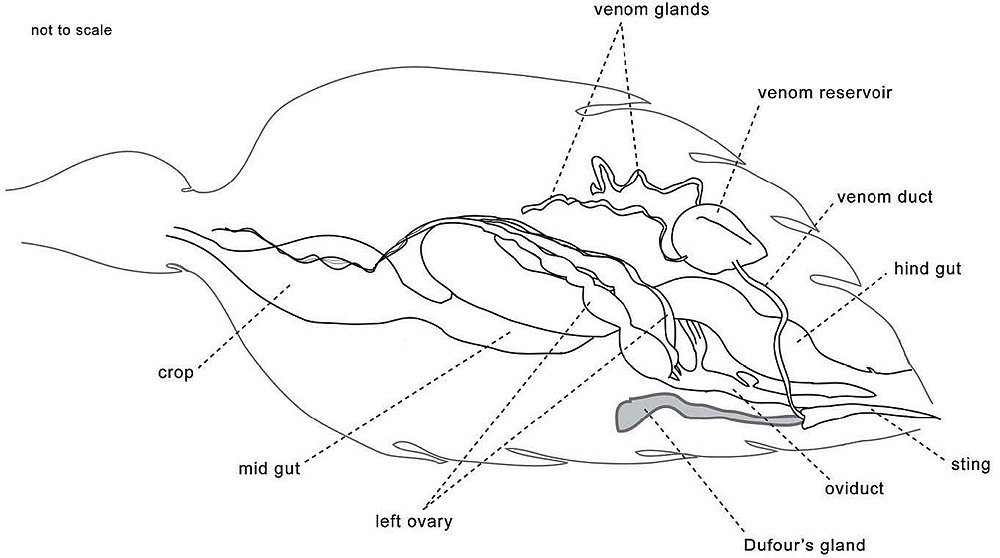
Having evidently found the source of the pheromone that guided ants to their forage, Wilson next wanted to determine the substance’s chemistry. “If successful, it would be a real breakthrough,” he writes, “an ant word identified in the ant Rosetta Stone.” A recent fortuitous development in chemical analysis, the work of chemists in the petroleum and tobacco industries, made that identification possible. It yoked two instruments that together could sort out small sam ples of mixed organic substances—substances such as the ants’ trail pheromone. One of the instruments was the gas chromatograph. It vaporized a compound and ran the resulting gas mixture through a column that separated the components. The other instrument was the mass spectrograph, which electrified the gaseous components the chromatograph produced, spread them out according to their mass, and displayed the resulting mass spectrum as a graph. That picture of distinct chemical peaks could then be compared with the spectra of known compounds to determine what the components were.
But a small sample for chemical and physical analysis was still much more than the bare speck of gland Wilson had extracted from a single fire ant. Each ant could supply, at best, a few micrograms—millionths of a gram. He needed milligrams—thousandths of a gram. To assay the fire-ant trail pheromone, then, Wilson would have to collect tens of thousands of fire ants.
Distilling 100,000 Fire Ants
He was no longer working alone. Anticipating his need for colleagues skilled in chemical analysis, he had recruited three chemists who had learned the new assay techniques to join him in the summer’s work: one from the Baylor College of Medicine in Houston, Texas; one from the University of Chicago; one a graduate research student at the Harvard Medical School.
Wilson knew where to find fire ants, by the hundreds of thousands, and he knew how to harvest them. In the late summer of 1958, he and his three chemist colleagues traveled south from Massachusetts to northeastern Florida, to a rural highway between Jacksonville on the coast and the Okefenokee National Wildlife Refuge inland. “Mound nests of fire ants abounded there,” Wilson writes, “next to ponds fed by quietly moving freshwater streams.” A typical mound might house as many as two hundred thousand ants, and there were mounds along the roadside, spaced apart about the length of a football field as far as the eye could see.
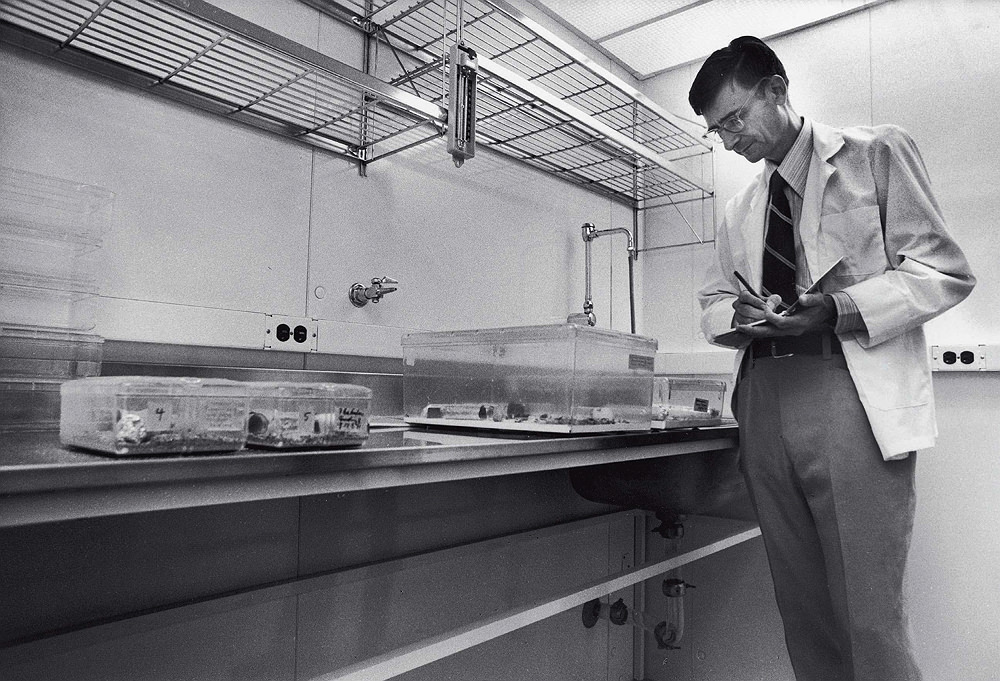
Wilson devised the Plexiglas habitat in which to observe ant populations within the laboratory.
Photograph by Hugh Patrick Brown/Getty Images
The red imported fire ant, a native of the South American floodplain, is adapted to protect its colonies from inundation, Wilson explains:
When the ants sense the approach of a flood from around and below them, they move to the surface of the nest, carrying with them all the young of the colony—the eggs, the grublike larvae, and the pupae—while nudging the mother queen upward as well. When the water reaches the nest chambers, the workers form a raft of their bodies. The whole colonial mass then floats safely downstream. When the ants contact dry land, they dissolve their living ark and dig a new nest.
By a rural Florida roadside on a summer day, the scientists broke out spades and buckets and began spading scoops of fire-ant mound into the buckets, which they dumped into a roadside pond. As the sand and dirt sank beneath the pondwater, the scrambling ants floated to the surface and assembled their living rafts. These the men scooped up with tea strainers and transferred into bottles they had partly filled with a low-boiling-point solvent, dichloromethane, ready to be distilled.
“Do what we can,” Wilson’s New England predecessor Ralph Waldo Emerson wrote in one of his essays, “summer will have its flies; if we walk in the woods we must feed mosquitoes.” If they collected fire ants, they must suffer the stings. “We came back [to Harvard] with the requisite one hundred thousand worker ants (roughly estimated, not counted!),” Wilson reminisces, “and my hands covered with itching welts from the stings of many angry ants.” His hands, and the hands of his colleagues. They never again volunteered to collect fire ants with Ed Wilson.
Now the chemists went to work teasing out the trail pheromone. They did that by steam-distilling batches of their solvent-dissolved ants, extracting the oily glandular secretions from the distillate with petroleum ether, another common laboratory solvent, and then separating the Dufour’s gland component with gas chromatography. Wilson tried laying trails with this extract to see if the ants responded as they had in his earlier experiments that used the crushed gland itself. They did, he reported in the journal Sciencein March 1959; the extract produced “trail-following responses of nearly comparable magnitude to those produced by [crushed Dufour’s] gland preparations.”
Wilson was thus the first investigator in the world to identify the basic system whereby ants communicate, an original and significant achievement. Along with that work in 1958 and 1959, he published more than 20 scientific and popular scientific papers in journals as diverse as Science (the premiere U.S. scientific journal), Psyche (a leading entomological journal), Pacific Insects, Natural History, and Scientific American. It isn’t surprising that he was awarded lifetime tenure and an associate professorship at Harvard in 1958, at the young age of 29. He’d earned the distinction, however much it made Jim Watson swear.
“Ceaseless and Futile Turmoil”
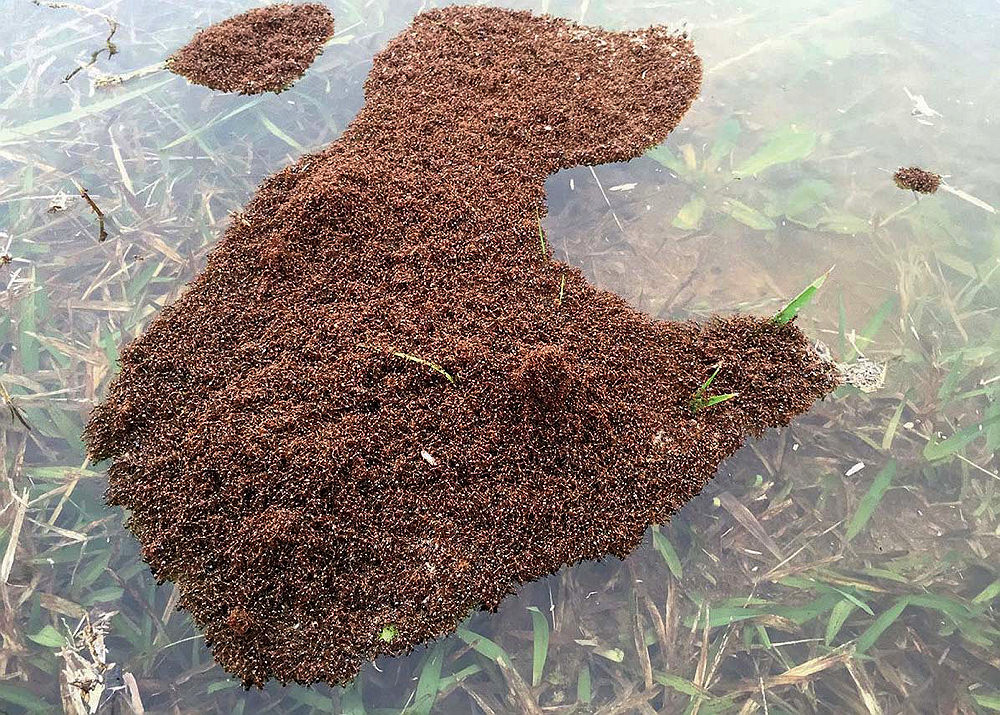
Fire ants, shoveled from nest into a pond, form a floating raft: the best way to capture a colony for transport to the lab.
TheCoz (own work) [CC BY-SA 4.0 (https://creativecommons.org/licenses/by-sa/4.0)], via Wikimedia Commons
Yet, despite his and his chemist colleagues’ painful work of fire-ant collection and pheromone extraction—not to mention the tedious repetitiousness of running the trail-following experiments six and more times each, measuring and counting, keeping track of ants no bigger than bread crumbs—the experimental trail trailed off. The Dufour’s gland substance proved to be “a relatively simple molecule,” Wilson writes, “—a terpenoid—and its complete molecular structure seemed within reach.” (Plants and insects produce a large variety of biologically active volatile oils known as terpenoids, many with intense odors, among them citronella, rose oil, and turpentine.) But the more the chemists purified the secretion, the less eagerly the ants responded. Something was awry.
“Was the pheromone an unstable compound?” Wilson asked himself. “Was the effect the result of not one but a mixture of substances being required?” He decided a mixture was a good possibility. His colleagues agreed, and, worse, saw no way to tease out the components of such a compound given the current state of their chemical-analysis technology. Wilson’s blunt conclusion, which speaks to what had been, after so much work, real pain: “We quit.”
Before they abandoned their research, they decided to sum it up in a report, a rare scientific paper describing a negative result, to establish a baseline for further investigation as chemical-analysis technology improved. The paper was sufficiently novel to win acceptance by the British journal Nature. Even there, although the trio acknowledged experiencing “some frustration” in the negative outcome of their attempts to identify the active trail substance, they saw a potential discovery in the fading of their pheromone. The fact that the purer extract failed to activate the ants, they wrote, “indicates the possibility that chemical instability may play a part in obliterating the trail substance after it has served its function. Wilson has previously suggested that this is accomplished by using a very volatile material for a trail substance.” A trail marker that continued to trigger ants to follow a trail after a food source had been collected would misdirect workers to a dead end.
And, in fact, in further studies, Wilson found that signal pheromones fade quickly unless other workers reinforce them—in the case of alarm signals that harvester ants spray into the air, within about 35 seconds. “The advantage to the ants of an alarm signal that is both local and short-lived,” he wrote in Scientific American in 1963, “becomes obvious when a [harvester ant] colony is observed under natural conditions. The ant nest is subject to almost innumerable minor disturbances. If the [sprayed] alarm spheres generated by individual ant workers were much wider and more durable, the colony would be kept in ceaseless and futile turmoil. As it is, local disturbances such as intrusions by foreign insects are dealt with quickly and efficiently by small groups of workers, and the excitement soon dies away.”
Not until 1981 did a research team—headed by Robert K. Vander Meer, the director of the U.S. Department of Agriculture’s fire-ant laboratory in Gainesville, Florida—tease out the complete mix of pheromones that combined in the ant Dufour’s gland. “The trail substance,” Wilson reports of that work, “…is not a single pheromone but a medley of pheromones, all released from the sting onto the ground. One attracts nestmates of the trail layer, another excites them into activity, and still another guides them through the active space [of vapor] created by the evaporating chemical streaks. All of the components need to be present to evoke the full response.” Wilson calls this combination “engineering by natural selection.” By not realizing its complexity, he concludes, “and thereby taking aim only at one of the components, we had failed to identify any of them.” Because evolution operates by modifying existing traits rather than inventing new ones, few phenomena in the natural world are simple. Yet Wilson’s contribution to the unraveling of the ant communication system was fundamental. “I was able,” he recalled much later, “to at least be the first to piece together how pheromones were being used as a means of communicating information between ants and within a colony.”
From Pheromones to “Proto-Sentences”
After Wilson’s 1958 discovery of the source of a trail pheromone component in the Dufour’s gland, a number of researchers armed with increasingly sophisticated instruments sorted out most of the many pheromones that ants secrete. By 1983, Robert Vander Meer could summarize reports of no fewer than nine different chemical compounds distilled from Dufour’s gland extract that contribute to trail marking alone, and in total, from just the Dufour’s gland, more than 50. By then, other pheromones produced elsewhere in the ant body had been identified as well: queen pheromones, brood pheromones, nestmate recognition pheromones, worker attractant pheromones, and more. Pheromones were ant language, a chemical dictionary of commands.
With them, Wilson would write, these highly social insects could even “put together pheromones with other odors to create ‘proto-sentences.’ A foraging worker, having encountered fire ants, rushes into its home nest with the equivalent of shouting Emergency, danger by spraying alarm pheromones, then Enemies by presenting the odor of fire ants it has acquired on its integument during a recent tussle, and then This way, follow me, as it turns and runs back along the odor trail it has just laid.”
Wilson’s fundamental experiments during this period of his career demonstrated that organismic biology could be as productive of discoveries as molecular biology and need not desiccate to a dry-bones molecular reductionism. At the same time, he had not forgotten his earlier work in the South Pacific and the questions it raised about how species colonize islands. Given the extremely complex and overlapping relationships among multiple species on continental land masses, might island colonization serve as a simpler model through which to study how evolution proceeds when species encounter each other and adapt? And since islands were numerous and variously isolated, and came in all sorts of sizes and shapes, couldn’t they serve for natural experiments as well, making it possible to test a theory under a variety of conditions? And wasn’t an island something more than a landmass surrounded by water? Mountains were insular; so were stands of forest surrounded by prairie; so were streams, caves, and tide pools; so, most urgently, were the formerly continuous natural habitats throughout the world increasingly broken up by human settlement. Learning how species were faring under such conditions would be important to preserving the natural world while the human world continued, seemingly inexorably, to encroach upon it.