“What a mess,” Sharad Ramanathan thinks, contemplating a group of cells growing in a glass dish. There are different cell types everywhere, the random “daughter cells” produced by a stem cell population. A mathematician and physicist by training, he wants to know what directs these cells’ fates—what caused the daughter cells to become heart or skeletal muscle or some other tissue across generations of cell division and differentiation. Tracing cell lineages is easy in the lab, helping him identify the factors that trigger specialization, says the Gund professor of neurosciences and of molecular and cellular biology. Ultimately, he wants to understand how a population of identical stem cells develop to form an aardvark or an ape.
This journey from cells to whole animals is the wondrous enigma at the heart of developmental biology. In principle, the process seems radically simple: just a handful of biochemical signaling families are the master regulators. But in practice, the many steps are astonishingly complex. The location, intensity, and timing of those developmental signals follow a choreography so intricate that it has been nearly impossible to recapitulate in the lab. In fact, scientists have spent years perfecting the recipe for directing a stem cell to become a specific cell type. Spurring the effort is its potential payoff: for example, painstaking work across more than a decade eventually induced stem cells to divide and differentiate into pancreatic beta cells, which secrete insulin. Those cells are being used experimentally to treat diabetes.
Given the difficulty of creating tissue types from stem cells, building physical structures, such as organs or the simplest body plan—of obvious use for experimental research, and potentially for therapeutic applications—has exceeded the scientists’ grasp. For instance, brain cells and primitive neural tissues, once a major focus of the Ramanathan lab, have been created from stem cells but remain many steps away from what would be considered a brain structure. Scientists can create “all the beautiful cell types of the brain,” Ramanathan explains, “but the layering and cortical structure is missing.”
If biologists could mimic early mammalian development with a reliable experimental system, they could study whole-body physiology, including the formation of organs and tissues; test drugs; seek cures for genetic diseases; and in the distant future, perhaps even grow human organs for transplant. About five years ago, Ramanathan resolved to create such a system.
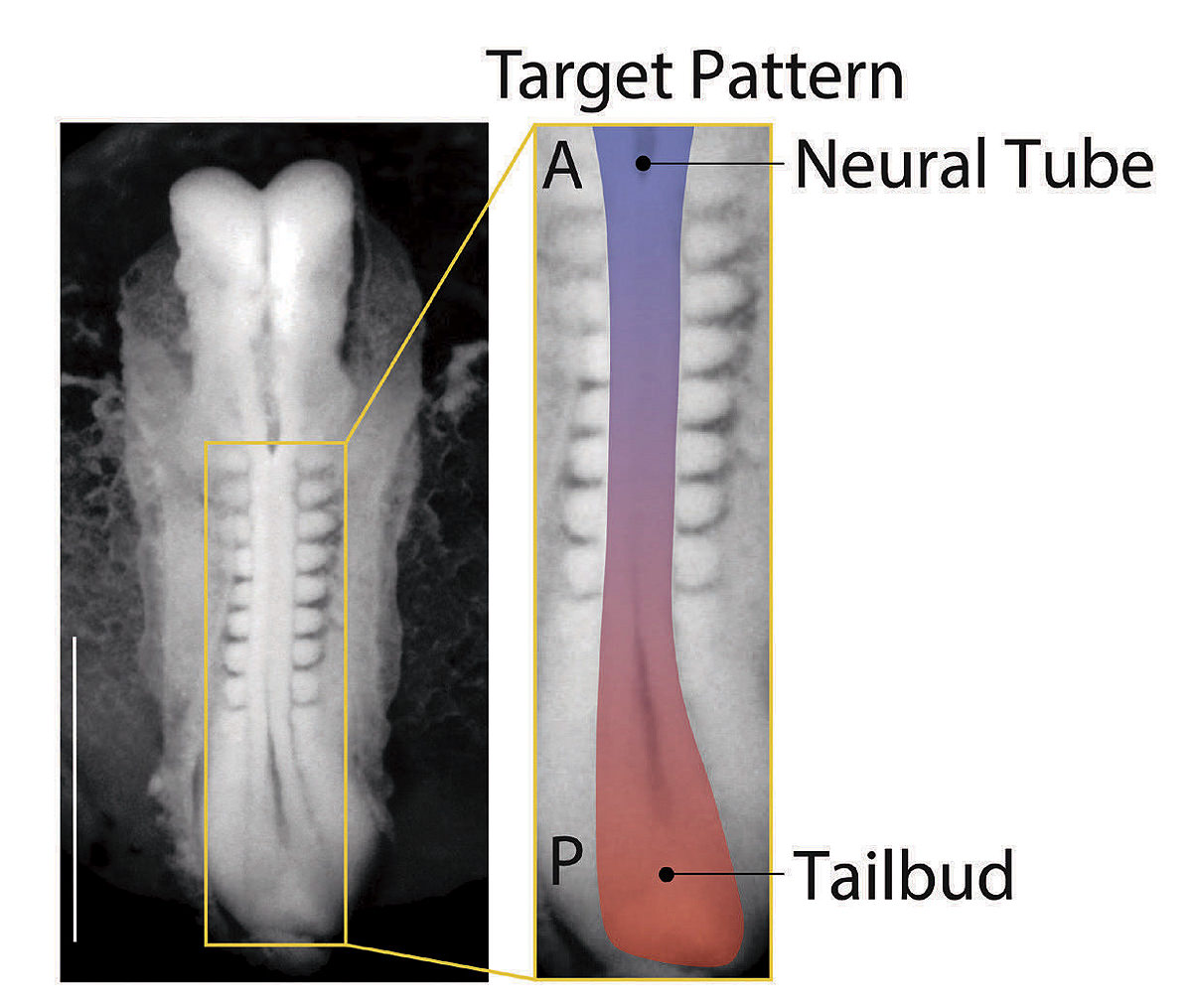
Colleagues describe Ramanathan as charming and mercurial. Math and physics enthralled him during his schooling in India. He enrolled as a Harvard doctoral student intending to study chemistry but soon switched to theoretical condensed matter physics. After earning his Ph.D. in 1997, he spent about a year at the Institute for Theoretical Physics in Santa Barbara before accepting a position at Bell Labs. There, in the rarified atmosphere of what was arguably the world’s leading industrial research and development enterprise, he was in the company of scientific luminaries, including some who would become Harvard colleagues: Cherry Murray, later the dean of the School of Engineering and Applied Sciences, and Wallace professor of applied physics Federico Capasso, renowned for developing lasers and optical devices that integrate quantum effects.
Ramanathan was told he could investigate anything—as long as the research was successful. This daunting charge, he says, triggered a “kind of existential crisis.” He decided to switch focus. Although he had never studied biology, he felt his quantitative skills could be usefully applied to problems in the life sciences. With Capasso’s backing (“Do whatever you want,” he recalls being told), Ramanathan enrolled in the famous yeast genetics course at Cold Spring Harbor Laboratory.
Yeast is a reliable, widely-used model system for studying the molecular biology of living organisms (other than bacteria). And the Cold Spring Harbor course has prepared many outstanding molecular biologists since its inception in 1970. Ramanathan proved adept at genetics research, and in 2005 returned to Harvard as a Bauer Fellow, one of several young and independent researchers chosen for that interdisciplinary postdoctoral program who had been recruited from fields such as physics, mathematics, chemistry, computer science, and engineering to investigate hard problems in biology. “I was looking for people like Sharad,” recalls Smith professor of molecular genetics Andrew Murray, then director of the Bauer program, who has remained a mentor to the younger scientist.
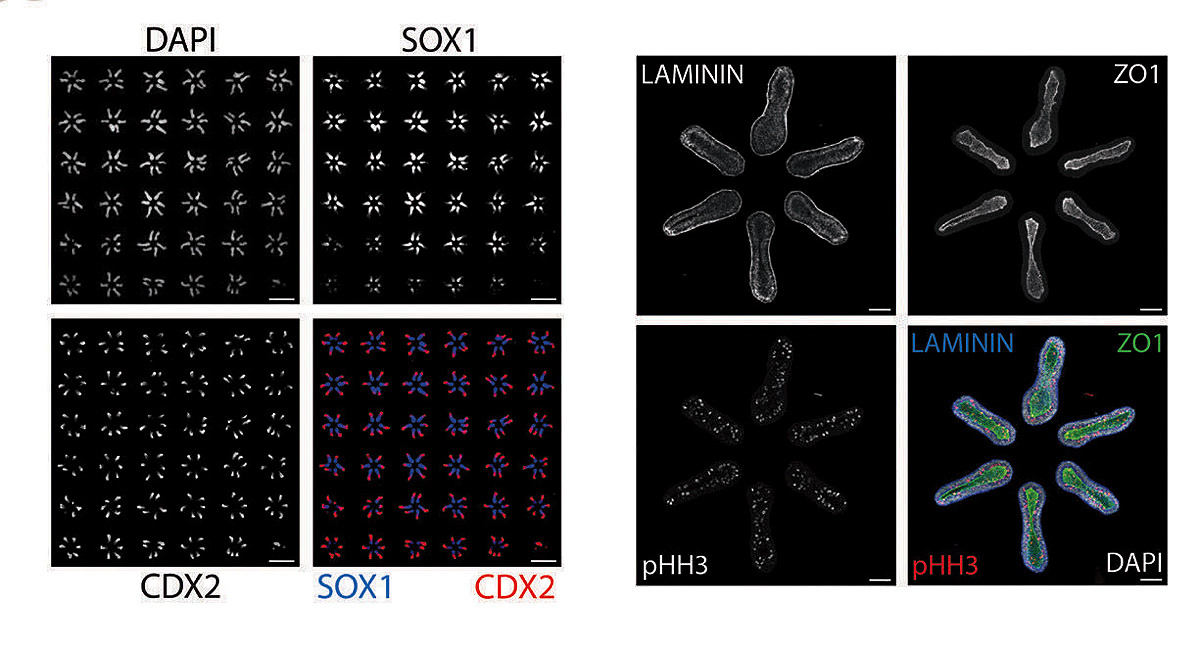
Ramanathan, still employed by Bell Labs, intended to continue his work with yeast. But in Cambridge, Doug Melton, the pioneering stem cell scientist, “took me under his wing,” Ramanathan recalls. Soon, he was working with mouse stem cells, and in 2007, Melton advised him to “burn up all the yeast and focus on stem cells.” Ramanathan’s analytical abilities—spanning multiple lines of life science, applied physics, and quantitative disciplines—equipped him with unusual potential to advance understanding of developmental biology using stem cells. He accepted a full-time position as a Harvard associate professor in 2008.
Ramanathan turned to this new field with gusto. “Some people were worried that I was jumping around too much,” he says—a legitimate concern. With mentorship from molecular and cellular biologist Catherine DuLac, now Morris University Professor, Ramanathan in 2013 joined the Seattle-based Allen Institute’s efforts to use human stem cells to understand cortical development. The cortex is believed to be the seat of higher cognition and is a principal characteristic of the human brain that distinguishes it from other animals. He began flying back and forth regularly between Cambridge and the West Coast. But by now he had two young children, and bicoastal treks proved an unsustainable regimen, as his wife (also a scientist) pointed out. So, in 2015, he consolidated his stem cell work in Cambridge.
There are many types of stem cells. Totipotent stem cells can theoretically develop into any tissue or organ in the animal from which they derive. Pluripotent stem cells can become many kinds of tissue—but never create a whole animal. Working now with pluripotent stem cells from both mice and humans, Ramanathan set an ambitious goal: to create a stem cell-based system for understanding developmental biology as tractable as those used in the study of far simpler yeast genetics. At this point, he decided to set aside his stem cell studies of human brain development. Creating human brain cells for study is one thing, and creating a human body plan and organs is another, but uniting them, he says, would raise ethical concerns. So he decided to focus on human physiology from the neck down.
He wanted “to do reproducible experiments and make it easy to perform manipulations,” explains Andrew Murray. “And if he couldn’t succeed in creating a fully functional experimental system within five or 10 years, he was going to do something else.”
Four years ago, Ramanathan, who is also professor of applied physics and of stem cell and regenerative biology, identified four basic problems that he and his lab colleagues had to solve; if they couldn’t, they agreed to shut down this line of research. The first three involve efficiently creating large numbers of genetically and physically identical copies of developing cell colonies in an easily managed system. The last involves sustaining growth long enough so that the cells can become tissues and acquire function.
In lab settings, says Ramanathan, only about one in a hundred clusters of pluripotent stem cells will reliably self-organize and differentiate—a substantial impediment to running large, comparative experiments. To identify the signals and genes that guide normal development and to understand what goes wrong in disease, one of the most important approaches to biological inquiry is to “see what goes wrong when we break something,” by knocking out a gene for example, he explains. But if the cells aren’t uniform at the outset, “You don’t know if you broke it or if it was already broken.” “To reduce costs,” he continues, researchers have to design a system that makes it easy to alter or remove genes so that all the cells in the dish undergo the same genetic change. The final and most challenging problem they face is how to sustain each colony’s growth. Absent a cardiovascular system to deliver oxygen or gut to process nutrients, the researchers’ experiments are unlikely to continue long enough to create functional tissues or the beginnings of organs.
Last year, in what Murray describes as a stunning breakthrough, Ramanathan’s lab essentially solved the first three problems. The key insight, he says, came from one of his students.
Giridhar “Giri” Anand and other graduate students in the lab had been suspending spherical colonies of pluripotent human stem cells in a three-dimensional gel on dozens of glass slides—about a hundred per slide. They encouraged the cells to grow by applying biochemical signals known to initiate differentiation into identifiable kinds of tissues. But the results appeared chaotic—until Anand noticed that the colonies of cells at the corner edges would repeatedly form an elongated shape. Although these fringe colonies appeared to have grown randomly, they were doing so in a persistent pattern from experiment to experiment. When Anand pointed this out, Ramanathan initially advised him not to focus on “edge cases” but rather, on what most of the colonies were doing. Anand began playing with the arrangement of the colonies in his spare time and realized that this affected their development in repeatable patterns. “It turned out,” he says, “that the cells were responding to their environment and their neighbors. They were actually communicating with each other.”
Making use of this insight, the lab staff began creating thousands of random samples using different arrangements of colonies and tracking the outcomes. Ramanathan took the resulting raw data, still chaotic, and applied statistical techniques to bear on the question of optimal arrangement: what spatial pattern would induce the largest number of cell colonies to differentiate and self-organize into tissues? Together, they discovered that the best arrangement would involve organizing six colonies into the shape of a hexagon. And they found they could fit a six-by-six array of these hexagonal units onto a single glass slide.
“This is absolutely revolutionary,” says Andrew Murray, “the ability to control how groups of stem cells develop. It really starts to mimic what happens in actual mammalian embryogenesis.”
In this configuration, 99 out of 100 pluripotent cell colonies reliably and reproducibly develop an anterior-posterior axis, then a front and a back side, and then a left and a right, with the beginnings of a spinal cord developing in the middle. DNA analysis of the cell types present include those that would go on to form the bones of the rib cage, as well as kidney and cardiac tissue, all in the expected positions. Patterning the correct physical structure of the tissues is critical because the cells continue to communicate with each other, guiding growth. “This is absolutely revolutionary,” says Andrew Murray, “the ability to control how groups of stem cells develop. It really starts to mimic what happens in actual mammalian embryogenesis. And that’s been a pretty serious Holy Grail for a large number of people. It’s the best of science, a truly spectacular achievement.”
One problem that the lab initially resolved to tackle remains: how to keep the cells growing to the point that tissues and organs acquire function. That obviously won’t happen on a glass slide, where the stem cell aggregates lack many of the fundamental systems, including a blood supply, that support life. But if one wanted to grow a human organ for transplant, how might that be possible? In experimental treatment at the University of Maryland Medical Center, physicians have transplanted pig hearts into human patients, who have survived for as long as two months with the transplants. But what if a human heart or liver could be grown from stem cell precursors in a chimeric pig or cow acting as host? Might that overcome problems of compatibility? There are too many unknowns to guess at the answer, and for now, Ramanathan calls the idea farfetched. But compared with engineering an artificial organ, he points out, “One of the most amazing things about biology is the ability of cells to self-organize. So, you might want to take advantage of that. Which is exactly what we have been trying to do.”
The Ramanathan lab’s advance will enable large-scale scientific inquiries into the intricacies of early development. “Biological systems are enormously complex,” he says. If thousands of identical groups of cells can be genetically altered or reprogrammed by a drug, that will enable new understanding into how cells self-assemble to create complex, integrated structures—and potentially lead to treatments for diseases like spina bifida or scoliosis that arise when things go awry. His group is already developing efficient, low-cost methods for testing the effects of large numbers of genetic alterations and drugs.
If allowed to develop further, the stem cell aggregates his group has created would likely begin to form limb buds, the next step in body plan development. But their potential for growth has been limited in specific ways by design. The lab modulates signaling molecules that promote differentiation to prevent the formation of the gut, for example, and the same approach is used to restrict body plan development to structures from the neck down. Ramanathan has adopted these limiting protocols in order to respect the internationally accepted and Harvard-imposed ethical guidelines for working with human stem cells. He also consults regularly with Harvard’s Embryonic Stem Cell Research Oversight committee (see “Ethics and Human Cells,” page 42), which reviews research across the University to ensure that it is ethically sound and conducted in pursuit of important scientific goals.
But the lab’s breakthrough has underscored that the limitations on such research will soon no longer be technical. Roya Huang, one of Ramanathan’s graduate students, remembers asking older students during her first year in the lab whether “people would ever be able to make an embryo in vitro?” And the answer was, “‘No way, that’s impossible.’ But it’s quickly becoming apparent that it’s very possible.” Within a couple of years, she predicts, “There won’t be technical limitations to a lot of things that we are ethically concerned about. I think it’s important to have those conversations pretty soon.”