Maryville, Tennessee, lies near the foothills of the Great Smoky Mountains, a range home to more tree species than exist in all of Europe. Benton Taylor grew up amidst this abundance, but as a boy, he barely noticed the plants. In the nearby national park, a family friend was raising—together with a menagerie of other mammals—a pair of bears orphaned as cubs. Taylor dreamed of studying these apex denizens of the forest, who forage at the top of the food chain. But as his education and understanding grew, his curiosity shifted to seed-dispersing animals, plants, and the soil and nutrients that sustain them: a trip down the trophic pyramid, driven by an appreciation of forests as ecological systems in which plants are primary producers. “Now I’ve half moved into the basement,” jokes the assistant professor of organismic and evolutionary biology, whose research encompasses the strategies plants use to obtain essential nutrients such as nitrogen, and how that, in turn, affects their ability to store another vital element with a global climate impact: carbon.
Will plants take up enough carbon in the future to offset the human activities driving climate change? This is a critical question, to which there aren’t yet good answers. Taylor aims to figure this out, developing new lines of inquiry into carbon flows between land and atmosphere, and experiments that delve into the interactions of microbes, warmer soil, and roots—all influences neither visible nor intuitive. There are no easy solutions to climate change, and much to learn, urgently.
Already, land-based plants absorb about one-third of the carbon dioxide (CO2) attributable to fossil-fuel burning worldwide. Understanding what might limit their ability to sequester carbon is therefore an enormously consequential line of research. Plants have absorbed more CO2 than anyone expected during the past four decades. Whether that mitigation of greenhouse gas will continue, increase, or reverse itself is a trillion-dollar question—and a complex one. The answer depends on worldwide trends in forest regeneration, especially in the mid-latitudes and the tropics. It depends on what happens to the vast quantities of carbon stored in Arctic soils during millennia past. And it also depends on whether plants, which use CO2 in photosynthesis, could grow faster in the future due to a fertilization effect as atmospheric carbon increases—and thus sequester more carbon.
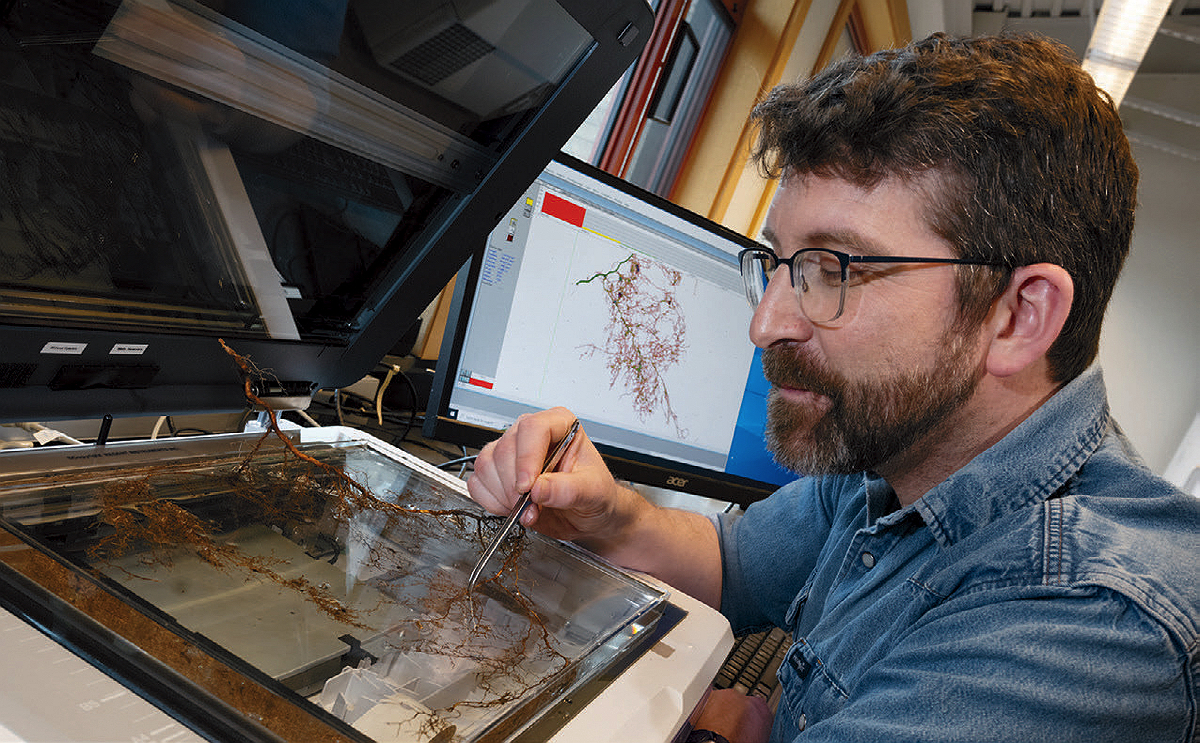
To understand this range of influences, Taylor runs experiments in ecosystems from the Alaskan Arctic to the tropical rainforests of Central America. In addition to the effects of elevated atmospheric CO2 on plant growth, his research encompasses changes in temperature, moisture, and severe weather patterns. He also studies global changes indirectly related to climate: in land use, the effects of industrial fertilizers and pollution, the impacts of pathogens and invasive species on native plants, and the influence of insect and animal herbivory—all with potential to affect the terrestrial biosphere. Plants are remarkably adaptable, Taylor observes. Already, the strategies they use for obtaining what they need to survive are shifting in response to these global changes.
Limiting the CO2 Dividend
Consider the idea that plants might grow faster in the presence of elevated atmospheric CO2, an appealing hypothesis in a rapidly warming world. But increased plant growth will become an important natural route of additional carbon sequestration only if tree growth is not constrained by some other factor such as limited availability of water, light, or (most likely) nitrogen, a key fertilizer. And increased plant growth won’t matter if other contingencies, such as the destruction of forests by fire, hurricanes of increasing frequency, or human hands, limit any CO2 dividend.
An example of the complexities that engage ecologists like Taylor when considering natural carbon sequestration is what happens when deer feed on hardwood saplings in a forest understory. At Black Rock Forest in the Hudson Valley, he and other scientists are simulating what would happen if a pathogen killed the oak trees. Pests and pathogens of prior generations decimated chestnuts and elms, and are currently laying waste to hemlock, ash, and beech forests. There are signs, says Taylor, that oaks may be vulnerable too. What would grow up in their place if the oaks succumbed, and how much carbon would the replacement species sequester? The answer may depend on what deer prefer to eat, so the Black Rock Forest experiment includes fenced areas where cervids are excluded.
Whitetail deer herbivory could change the capacity of northern hardwood forests to sequester CO2.
“When the canopy opens up,” Taylor explains, “it frees a lot of additional resources, primarily light, but also other things that the adult trees aren’t taking up from the soil anymore. And there’s this rush at the seedling layer to take advantage of those resources. That initial competition is really important,” he emphasizes. “Which of these species will extract more resources from the soil to grow a little bit taller, overtop its competitors, and win the light competition?” At first glance, that might appear to be a key question. “But that all goes out the window if a deer comes along and munches the top off,” he cautions. Deer even give invasive plants an edge in a recovering forest, he says, because the animals prefer to browse on native species. “Suffice it to say that whitetail deer herbivory is a huge driver of understory competitive dynamics”—one that could change the future composition of northern hardwood forests and, ultimately, their capacity to sequester CO2.
As it happens, there is a link between what makes plants grow faster and what deer prefer to eat. “Typically, what makes a leaf more nutritious”—and thus appealing to deer, he says, —“is how much nitrogen it has in it.” Nitrogen “tends to be the most limiting resource for most organisms, especially plants.” And it is likely to be a key determinant of how much carbon plants will store.
A Rainforest Revelation
Taylor’s interest in nitrogen and other “basement” soil nutrients came about gradually: “No little boy grows up fantasizing about nitrogen fixation,” he notes wryly. But his holistic, ecosystem-scale take on what’s happening in the woods does owe something to his upbringing. His mother and father met while working for the National Park Service. Although they changed occupations when he and his brother were born, the family went backpacking most weekends, and his parents’ familiarity with the mountains and their understanding of the natural world left a strong impression. From the informal classroom of the Smokies, Taylor went on to study biology at Maryville College, across the street from his family home, then pursued a master’s degree at Clemson.
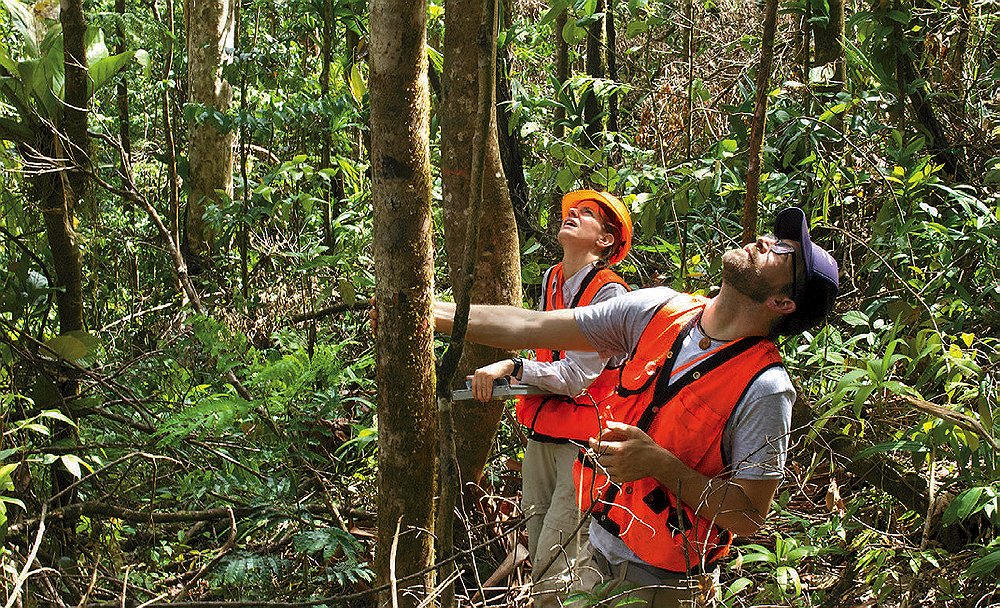
His first field research experience there changed his perspective on how ecosystems work. On the Caribbean island of Dominica, he began studying agoutis, rodents that look like hamsters but are the size of house cats. Agoutis disperse seeds across the forest floor much as North American squirrels do, Taylor explains, moving and burying them—activity that has “important implications for the distribution of species anywhere agoutis live.” During this research, Taylor spent a lot of time sitting by himself in the rainforest thinking and observed that “most of the behavior of the animals that live in a terrestrial ecosystem is driven by the resources that the plant community provides. I still think animals are cool, but that’s when I realized that if you really want to understand the system at its base, you need to focus on the plants.”
Doctoral research at Columbia shifted his perspective further earthward, to the environmental factors that control the input of nitrogen into ecosystems, and the question of whether they might mediate growth. In Costa Rica, he undertook an experiment to see whether planting nitrogen-fixing species in a regenerating rainforest would accelerate tree growth and thus carbon capture. His intuition was that it would, but that proved incorrect. Taylor stresses that a continuing theme in his lab’s research has been that “in nature, just because something makes sense, doesn’t make it true.” Instead, he found the “exact opposite effect”: the more nitrogen fixers there were in a hectare of forest, the slower it grew. “Although they provide nitrogen to the system,” nitrogen fixers turned out to be such strong competitors that their negative effects on other kinds of neighboring trees, deprived of resources such as light, slowed the regrowth of the forest overall.
“It is easy to assume that more above-ground tree growth should equal more soil carbon storage,”
Another counterintuitive example concerns carbon sequestration. Photosynthesizing trees convert CO2 into sugars (carbon compounds) that are used to build biomass. Then, as leaves fall or the trees die, much of that biomass is incorporated into soil, where it can be stored for long periods of time; that’s the general process by which fossil fuel reserves formed millions of years ago. “It is easy to assume that more above-ground tree growth should equal more soil carbon storage,” Taylor continues. “But very often, we do not find that to be true.” Instead, there’s a tradeoff between visible growth and soil carbon accumulation. “We are investigating a lot of plausible reasons why that might be the case.” For instance, environments with lots of moisture enable lots of above-ground growth. But they also accelerate the process by which microbes break down leaf matter that has fallen to the forest floor (releasing the stored carbon), so periods of drought, when trees grow less, can nevertheless lead to more carbon sequestration. His larger point: “In terrestrial global change ecology, many of these initial intuitive hypotheses make perfect sense, but very often are just not borne out by data.”
Subterranean Symbioses
Why the varied and surprising results? Taylor suspects that some of the answers lie underground, in the symbiotic relationships that plant roots form with microbes. After he arrived at Harvard in 2020 (following a postdoctoral fellowship at the Smithsonian Environmental Research Center near Annapolis) and set up a lab at the Arnold Arboretum’s Weld Hill Research Building, his focus on the hidden, subterranean interactions of plants intensified. His research tracks several types of interactions among roots, soil, and soil microbes.
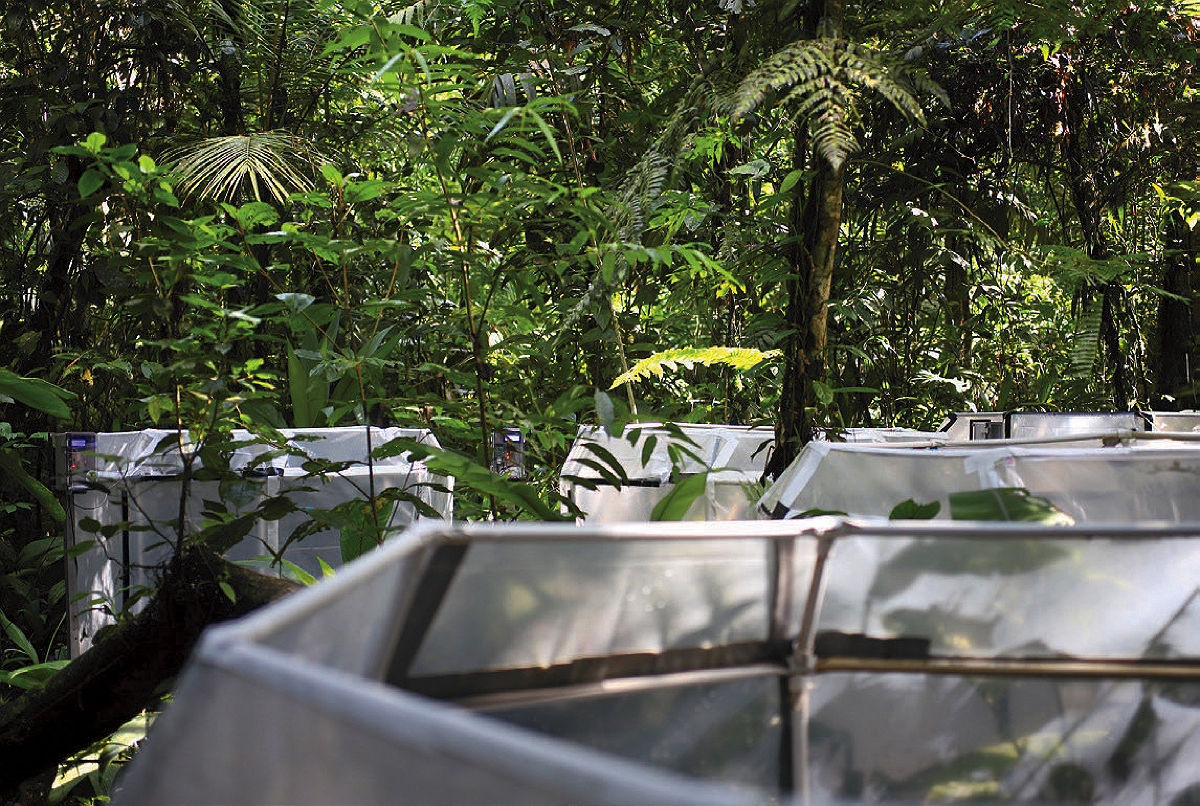
One set of experiments involves symbiotic relationships between trees and fungi. “The fungus pulls nutrients and water out of the soil and provides it to the tree,” Taylor explains. “And in exchange, the tree provides carbon to the fungus.” Two major groups of fungi form mycorrhizal (symbiotic) relationships with tree roots where these exchanges take place. One group is much better at providing nitrogen, the other at providing phosphorous. Each associates with distinct species of trees. And each imposes different costs on the tree in terms of the carbon required to sustain this mutualistic relationship. They represent completely different strategies for marshaling resources in the soil to support a tree’s growth, he explains: “There’s a lot of evidence that the carbon and nutrient cycles in a forest” that is dominated by higher latitude species such as conifers, beeches, and oaks, which associate with one type of fungus, “are very different than those in a forest” dominated by the other type, which is more common in tropical rainforests.
Although the two groups of fungi and their partner species often grow in different environments, the northern temperate zone is the place on earth where the tree species supported by these distinct strategies commingle, affording Taylor the opportunity to compare their responses to different climatic conditions. In a 50-hectare plot at the Harvard Forest in Petersham, Massachusetts, where the growth of approximately 16,000 trees is measured every 5 years as part of a global network of monitored plots, he and other researchers, including his graduate student Carina Berlingeri, can study the competitive dynamics of the two approaches.
“We have this beautiful mixing of species,” he says, “that lets us compare the growth rates” of trees associated with each strategy. They can even compare growth rates in different microenvironments, such as swamps versus uplands. And in nearby experiments where the soil is heated—which originally enabled researchers to determine that elevated temperatures dramatically change the composition of microbial communities that live there—Taylor is probing whether higher temperatures change the strategies that roots and fungi use to forage for soil resources (a critical variable as climate elevates the warmth of the air and the soil).
And comparisons between the two types of fungal symbiosis are also being explored under controlled laboratory conditions at the Arboretum, he notes: “We’re pairing that [Harvard Forest research] with greenhouse work,” where growth chambers provide tightly controlled environments to simulate future global climate change scenarios. That, he says, enables “informed predictions for how these competitive dynamics are going to change in the future.” Crucially, these smaller-scale projects enable the researchers to run their experiments far more quickly than observing nature run its course: within about six months, the seedlings start crowding each other. “What wins that initial competition for light, what lets one species grow taller than another,” Taylor says, suggests which trees will dominate forests of the future.
The Sleeping Giant
At the Weld Hill greenhouses, Taylor is conducting a related experiment that pits the two types of tree-fungus associations directly against each other. Cottonwood trees, rare in the Northeast but common along streams in the West, are unusual in that they can form associations with either of the two groups of fungi. “Comparing oaks and maples, are you really measuring the costs and benefits of the different mycorrhizal types,” he asks, “or are you just seeing intrinsically different things about maples versus oaks? Cottonwoods take the species quandary out of the mix.” The team is using isotopically labeled nutrients that will allow them to track the exchange of nitrogen, phosphorous, and carbon between the plants and their fungal networks under changing global conditions of temperature, moisture, and light.
In the lab itself, roots are being suspended in liquid, disentangled, and separated by size and function prior to scanning. Scientists will use software to analyze the images of these curving and intricately branched networks to calculate an overall length, were the roots all straight. That’s one way of measuring a plant’s growth, but “a lot of what we do in this lab,” Taylor continues, “is take nature”—leaves, roots, and stems—“dry it, grind it into powder, and then burn it in various ways to analyze it.” Grinders are the lab’s workhorses. There’s a tiny tabletop wood chipper, and another that shakes ball bearings in a canister to powder plant material. “If you want to know how much carbon or nitrogen is in leaves or roots, you place the powder in a tinfoil cup, and crush it into a tiny ball,” he explains. A conveyor belt carries the ball into a desktop furnace that incinerates the entire package, measuring only the nitrogen, carbon, and other plant components.
This type of analysis is yielding new information about how much carbon trees are sending to their microbial partners below ground. “Think about how many roots are under our feet,” Taylor says emphatically. “It’s a huge pathway for moving carbon captured during photosynthesis into the soil.”
But balancing optimism about the capacity of ecosystems worldwide to absorb excess carbon is what’s happening in the Arctic. “The warming tundra represents a sleeping giant in the global carbon cycle,” he says. “There is a massive amount of carbon stored there that we’ve largely been able to ignore because it has been frozen for thousands of years.” If the permafrost atop this grassland thaws, that will expose the carbon trapped there to microbes. “They are constantly, like little Pac-Men, eating through the carbon in the soil. And when they eat a carbon-containing molecule,” he warns, “it is released to the atmosphere as CO2 or methane. That is a huge concern for climate scientists, particularly those that work in the Arctic.” Taylor’s research there with Ph.D. student Calvin Heslop focuses on alder, a nitrogen-fixing shrub that thrives in poor soils, and seems to open nearby ground to colonization by other plant species. The shrub also traps and shades snow, which paradoxically insulates and warms the tundra like a blanket in the frigid Arctic winter. “We’re trying to understand the carbon and nitrogen impacts of these alder shrubs and track changes in their prevalence by region,” he explains, “so we can start to predict where their effects are going to have the biggest impact in the future.”
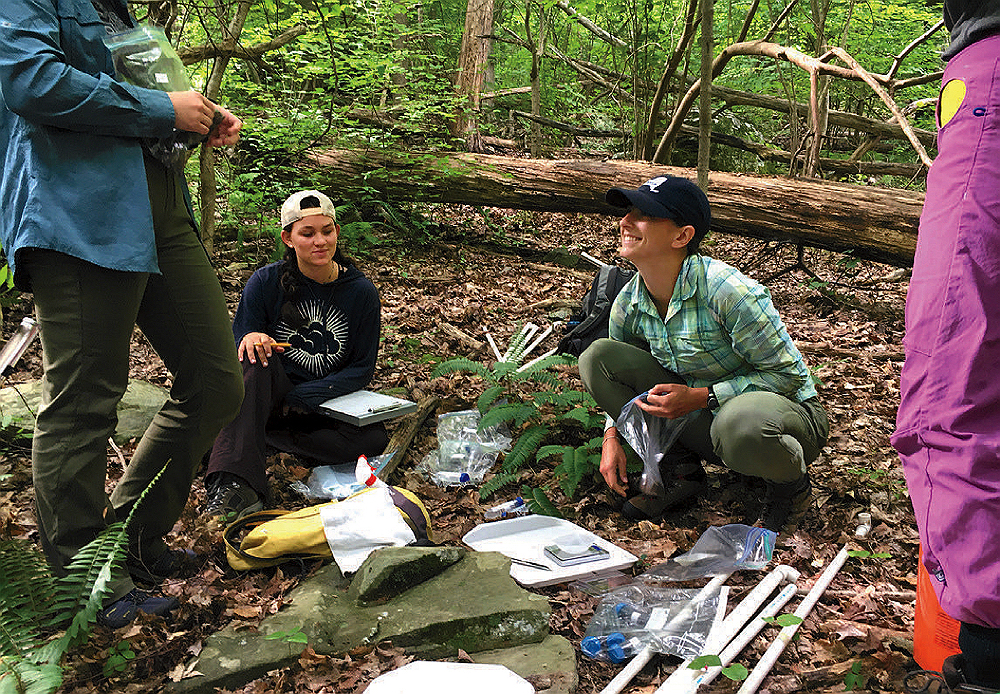
Expect surprises, Taylor says. The earliest theories about how ecosystems would respond to rising CO2 predicted an initial pulse of extra growth that would be quickly capped by nitrogen limitation. “That certainly has played out in many cases experimentally,” he acknowledges, “but I have worked on several projects where that has not been the case”—at least not during the expected timescale. “Plants are quite good at changing their resource acquisition strategies to sustain growth under new conditions.”
Might human intervention—or restraint—increase the natural uptake of CO2? Could planting a trillion trees, for example, slow global warming? Although skeptical of that particular strategy, Taylor says that understanding natural ecosystems’ ability to slow—or accelerate—climate change is crucial. Planting trees and finding different ways of improving soil carbon storage are all “good ideas, very close to the work that my lab does. But in the end, they’re only going to get us part of the way there. As far as I can tell,” he says, “there are no plausible and ethical solutions to our predicament that don’t involve cutting emissions. I don’t think…that this extra growth from elevated CO2 will go on forever.”